Sense: Difference between revisions
Iridescent (talk | contribs) m →Magnetoception: Cleanup and typo fixing, typo(s) fixed: north-south → north–south |
Adding, citing, and reorganizing reflecting the views of modern psychobiology and neuroscience; migrating some topic specific content to Perception Tag: nowiki added |
||
Line 1: | Line 1: | ||
{{About|the |
{{About|the cognitive process of sensation||}} |
||
{{Redirect|Five senses}} |
|||
{{short description|Physiological capacity of organisms that provides data for perception}} |
{{short description|Physiological capacity of organisms that provides data for perception}} |
||
{{Multiple issues| |
|||
{{more citations needed|date=January 2019}} |
|||
{{original research|date=January 2019}}}} |
|||
[[File: Five senses.jpg|thumb|318x318px|Five Aristotelian senses and their respective sensory organs inherent among ''[[Homo sapiens]]'']] |
|||
[[File:Claesz., Pieter - Still Life with Musical Instruments - 1623.jpg|thumb|270px|An [[allegory]] of five senses. Still Life by [[Pieter Claesz]], 1623. The painting illustrates the senses through musical instruments, a compass, a book, food and drink, a mirror, [[incense]] and an open perfume bottle. The [[tortoise]] may be an illustration of touch or an allusion to the opposite (the tortoise isolating in its shell).]] |
|||
The physical process during which [[Sensory nervous system|sensory systems]] respond to [[Stimulus (physiology)|stimuli]] and provide data for [[perception]] is called '''sensation'''.<ref name="wol" /> During sensation, sense organs engage in stimulus collection and [[Transduction (physiology)|transduction]].<ref name="pri">Privitera, A. J. (2020). Sensation and perception. In R. Biswas-Diener & E. Diener (Eds), Noba textbook series: Psychology. Champaign, IL: DEF publishers. Retrieved from http://noba.to/xgk3ajhy</ref> Sensation is often differentiated from the related and dependent concept of ''perception'', which processes and integrates sensory information in order to give meaning to and understand detected stimuli, giving rise to subjective perceptual experience, or [[qualia]].<ref name="sch">{{cite book|last=Schacter|first=Daniel|url=https://archive.org/details/psychology0000scha|title=Psychology|publisher=Worth Publishers|year=2011|url-access=registration}}</ref> Sensation and perception are central to, and precede, almost all aspects of [[cognition]], [[behavior]], and [[thought]].<ref name="wol" /> |
|||
A '''sense''' is a [[physiological]] capacity of [[organism]]s that provides data for [[perception]]. The senses and their operation, classification, and theory are overlapping topics studied by a variety of fields, most notably [[neuroscience]], [[cognitive psychology]] (or [[cognitive science]]), and [[philosophy of perception]]. The [[nervous system]] has a specific [[sensory nervous system]], and a sense organ, or sensor, dedicated to each sense. |
|||
[[File:SensoryProcessing.png|alt=|thumb|440x440px|Sensation consists of signal collection and transduction]] |
|||
In organisms, [[Sensory organ|a sensory organ]] consists of a group of related [[Sensory neuron|sensory cell]]<nowiki/>s that respond to a specific type of [[Stimulus (physiology)|physical stimulus]]. Via [[Cranial nerves|cranial]] and [[Spinal nerve|spinal nerves]], the different types of [[Sensory neuron|sensory receptor cells]] ([[mechanoreceptors]], [[Photoreceptor cell|photoreceptors]], [[chemoreceptor]]<nowiki/>s, [[thermoreceptor]]<nowiki/>s) in sensory organs [[Transduction (physiology)|transduct]] sensory information from sensory organs towards the [[central nervous system]], to the [[Sensory cortex|sensory cortices]] in the [[Cerebral cortex|brain]], where sensory signals are further processed and interpreted (perceived).<ref name="wol" /><ref name=":0" /><ref name="mcb">{{Cite book|url=https://archive.org/details/molecularcellbio00lodi|title=Molecular cell biology|date=2000|publisher=W.H. Freeman|others=Lodish, Harvey F.|isbn=0716731363|edition=4th|location=New York|oclc=41266312|url-access=registration}}</ref> Sensory systems are often divided into external (exteroception) and internal ([[interoception]]) sensory systems, or '''senses'''.<ref name=":1">{{Cite book|last=Campbell, Neil A.|url=http://worldcat.org/oclc/1017000156|title=Biology.|date=2017|publisher=Pearson Education UK|isbn=978-1-292-17044-2|oclc=1017000156}}</ref><ref>{{Cite book|last=Tsakiris, Manos, editor. Preester, Helena de, editor.|url=http://worldcat.org/oclc/1036733582|title=The interoceptive mind : from homeostasis to awareness|isbn=0-19-881193-4|oclc=1036733582}}</ref> Sensory modalities or submodalities refer to the way sensory information is [[encoded]] or [[Transduction (physiology)|transduced]].<ref name=":0" /> [[Multisensory integration|Multimodality]] integrates different senses into one unified perceptual experience. For example, information from one sense has the potential to influence how information from another is perceived.<ref name="pri" /> Sensation and perception are studied by a variety of related fields, most notably [[psychophysics]], [[neurobiology]], [[cognitive psychology]], and [[cognitive science]].<ref name="wol" /> |
|||
[[Human|Humans]] have a multitude of sensory systems. Human external sensation is based on the sensory organs of the [[Human eye|eyes]], [[Human ear|ears]], [[Human skin|skin]], [[inner ear]], [[Human nose|nose]], and [[Human mouth|mouth]]. The corresponding sensory systems of the [[Visual system|visual system (sense of vision)]], [[Auditory system|auditory system (sense of hearing)]], [[Somatosensory system|somatosensory system (sense of touch)]], [[Vestibular system|vestibular system (sense of balance)]], [[Olfactory system|olfactory system (sense of smell)]], and [[Gustatory system|gustatory system (sense of taste)]] contribute, respectively, to the [[Perception|perceptions]] of [[Visual perception|vision]], [[hearing]], [[touch]], [[Equilibrioception|spatial orientation]], [[Olfaction|smell]], and [[Flavor|taste (flavor)]].<ref name="pri" /><ref name="wol" /> Internal sensation, or interoception, detects stimuli from internal organs and tissues. A large number of internal sensory and perceptual systems exists in humans, including [[Proprioception|propioception (body position)]] and [[Nociception|nociception (pain)]]. Further internal [[Chemoreceptor|chemoreception]] and [[Osmoreceptor|osmoreception]] based sensory systems lead to various perceptions, such as [[Hunger (physiology)|hunger]], [[thirst]], [[suffocation]], and [[nausea]], or different involuntary behaviors, such as [[vomiting]].<ref name=":1" /><ref>{{Cite book|last=Tsakiris, Manos, editor. Preester, Helena de, editor.|url=http://worldcat.org/oclc/1036733582|title=The interoceptive mind : from homeostasis to awareness|isbn=0-19-881193-4|oclc=1036733582}}</ref><ref>{{Cite journal|last=Khalsa|first=Sahib S.|last2=Lapidus|first2=Rachel C.|date=2016-07-25|title=Can Interoception Improve the Pragmatic Search for Biomarkers in Psychiatry?|url=http://dx.doi.org/10.3389/fpsyt.2016.00121|journal=Frontiers in Psychiatry|volume=7|doi=10.3389/fpsyt.2016.00121|issn=1664-0640}}</ref> |
|||
Humans have a multitude of sensors. Sight ([[Visual perception|vision]], visual sense), hearing ([[Hearing|audition]], auditory sense), taste ([[taste|gustation]], gustatory sense), smell ([[olfaction]], olfactory sense), and touch ([[Somatosensory system|somatosensation]], somatosensory sense) are the five traditionally recognized senses. The ability to detect other stimuli beyond those governed by these most broadly recognized senses also exists, and these [[sensory modalities]] include temperature ([[thermoception]]), kinesthetic sense ([[proprioception]]), pain ([[nociception]]), balance ([[equilibrioception]]), vibration ([[mechanoreceptor|mechanoreception]]), and various internal stimuli (e.g. the different [[chemoreceptor]]s for detecting [[salt]] and [[carbon dioxide]] concentrations in the blood, or sense of [[hunger]] and sense of [[thirst]]). However, what constitutes a sense is a matter of some debate, leading to difficulties in defining what exactly a distinct sense is, and where the borders lie between responses to related stimuli. |
|||
Nonhuman [[Animal|animals]] experience sensation and perception, with varying levels of similarity to and difference from humans and other animal species. For example, [[Mammal|mammals]], in general, have a stronger sense of smell than humans. Some animal species lack one or more human sensory system analogues, some have sensory systems that are not found in humans, while others process and interpret the same sensory information in very different ways. For example, some animals are able to detect [[Electrical field|electrical]]<ref>{{cite conference|last=Kalmijn|first=A.J.|date=1988|title=Detection of Weak Electric Fields|conference=International Conference on the Sensory Biology of Aquatic Animals|publisher=Springer Nature Switzerland AG|doi=10.1007/978-1-4612-3714-3|isbn=978-1-4612-8317-1|editor-last4=Tavolga|editor-last3=Popper|editor-first3=Arthur N.|editor-last2=Fay|editor-first4=William N.|book-title=Sensory Biology of Aquatic Animals|editor-first1=Jelle|editor-last1=Atema|editor-first2=Richard R.}}</ref> and [[Magnetic field|magnetic fields]],<ref>{{cite journal|last1=Walker|first1=Michael M.|last2=Dennis|first2=Todd E.|last3=Kirschvink|first3=Joseph L.|date=December 2002|title=The magnetic sense and its use in long-distance navigation by animals|journal=Current Opinion in Neurobiology|volume=12|issue=6|pages=735–744|doi=10.1016/S0959-4388(02)00389-6|pmid=12490267}}</ref> [[air moisture]]<ref name=":2" />, or [[polarized light]]<ref>{{Citation|last=Cronin|first=T.W.|title=Polarized-Light Vision in Land and Aquatic Animals|date=2010|url=http://dx.doi.org/10.1016/b978-0-12-374203-2.00164-0|work=Encyclopedia of the Eye|pages=461–468|publisher=Elsevier|isbn=978-0-12-374203-2|access-date=2020-03-04}}</ref>, while others sense and perceive through alternative systems, such as [[Animal echolocation|echolocation]].<ref>{{Cite book|last=Fenton, M. Brock. editor. Grinnell, Alan D. editor. Popper, Arthur N. editor. Fay, Richard R. editor.|url=http://worldcat.org/oclc/1127113751|title=Bat Bioacoustics|isbn=1-4939-3527-5|oclc=1127113751}}</ref><ref>{{Cite journal|last=Kyhn|first=L. A.|last2=Jensen|first2=F. H.|last3=Beedholm|first3=K.|last4=Tougaard|first4=J.|last5=Hansen|first5=M.|last6=Madsen|first6=P. T.|date=2010-05-14|title=Echolocation in sympatric Peale's dolphins (Lagenorhynchus australis) and Commerson's dolphins (Cephalorhynchus commersonii) producing narrow-band high-frequency clicks|url=http://dx.doi.org/10.1242/jeb.042440|journal=Journal of Experimental Biology|volume=213|issue=11|pages=1940–1949|doi=10.1242/jeb.042440|issn=0022-0949}}</ref> Recently, it has been suggested that [[Plant|plants]] and [[artificial agents]] may be able to detect and interpret environmental information in an analogous manner to animals.<ref name=":3" /><ref name=":4">{{Citation|last=Les|first=Zbigniew|title=Machine Perception—Machine Perception MU|date=2019-08-02|url=http://dx.doi.org/10.1007/978-3-030-24070-7_2|work=Machine Understanding|pages=9–44|publisher=Springer International Publishing|isbn=978-3-030-24069-1|access-date=2020-03-04|last2=Les|first2=Magdalena}}</ref><ref name=":5">{{Cite book|last=Serov, Alexander|url=http://worldcat.org/oclc/1106181879|title=Subjective Reality and Strong Artificial Intelligence|date=2013-01-27|oclc=1106181879}}</ref> |
|||
Other animals also have receptors to sense the world around them, with degrees of capability varying greatly between species. Humans have a comparatively weak sense of smell and a stronger sense of sight relative to many other [[mammals]] while some animals may lack one or more of the traditional five senses. Some animals may also intake and interpret sensory [[Stimulus (psychology)|stimuli]] in very different ways. Some species of animals are able to sense the world in a way that humans cannot, with some species able to sense [[electrical field|electrical]]<ref>{{cite conference|conference=International Conference on the Sensory Biology of Aquatic Animals|book-title=Sensory Biology of Aquatic Animals|title=Detection of Weak Electric Fields|last=Kalmijn|first=A.J.|editor-last1=Atema|editor-first1=Jelle|editor-last2=Fay|editor-first2=Richard R.|editor-last3=Popper|editor-first3=Arthur N.|editor-last4=Tavolga|editor-first4=William N.|publisher=Springer Nature Switzerland AG|date=1988|doi=10.1007/978-1-4612-3714-3|isbn=978-1-4612-8317-1}}</ref> and [[magnetic field]]s,<ref>{{cite journal|journal=Current Opinion in Neurobiology|title=The magnetic sense and its use in long-distance navigation by animals|last1=Walker|first1=Michael M.|last2=Dennis|first2=Todd E.|last3=Kirschvink|first3=Joseph L.|volume=12|issue=6|pages=735–744|date=December 2002|doi=10.1016/S0959-4388(02)00389-6|pmid=12490267}}</ref> and detect [[water pressure]] and currents.{{Citation needed|date=March 2019}} |
|||
== |
== Definitions == |
||
{{Main|Psychophysics}} |
|||
[[File:Peacock served in full plumage (detail of BRUEGHEL Taste, Hearing and Touch).jpg|thumb|Detail of ''The Senses of Hearing, Touch and Taste'', [[Jan Brueghel the Elder]], 1618]] |
|||
A broadly acceptable definition of a sense would be "A system that consists of a group of sensory cell types that responds to a specific physical phenomenon, and that corresponds to a particular group of regions within the brain where the signals are received and interpreted." There is no firm agreement as to the number of senses because of differing definitions of what constitutes a sense. |
|||
=== Sensory modalities === |
|||
The senses are frequently divided into exteroceptive and interoceptive: |
|||
{{Main|Sensory modalities}} |
|||
* ''Exteroceptive senses'' are senses that perceive the body's own position, motion, and state, known as ''proprioceptive senses''. External senses include the traditional five: sight, hearing, touch, smell and taste, as well as [[thermoception]] (temperature differences) and possibly an additional weak [[magnetoception]] (direction).<ref name="Voustianiouk 2000">{{cite journal | vauthors = Voustianiouk A, Kaufmann H | title = Magnetic fields and the central nervous system | journal = Clinical Neurophysiology | volume = 111 | issue = 11 | pages = 1934–5 | date = November 2000 | pmid = 11068225 | doi = 10.1016/S1388-2457(00)00487-9 }}</ref> Proprioceptive senses include [[nociception]] (pain); [[equilibrioception]] (balance); [[proprioception]] (a sense of the position and movement of the parts of one's own body). |
|||
Sensory modality refers to the way that information is encoded, which is similar to the idea of [[Transduction (physiology)|transduction]]. The main sensory modalities can be described on the basis of how each is transduced. Listing all the different sensory modalities, which can number as many as 17, involves separating the major senses into more specific categories, or submodalities, of the larger sense. An individual sensory modality represents the sensation of a specific type of stimulus. For example, the general sensation and perception of touch, which is known as somatosensation, can be separated into light pressure, deep pressure, vibration, itch, pain, temperature, or hair movement, while the general sensation and perception of taste can be separated into submodalities of [[sweet]], [[salty]], [[sour]], [[bitter]], and [[umami]], all of which are based on different chemicals binding to [[Sensory neuron|sensory neurons]].<ref name=":0">{{Cite book|last=|first=|url=http://cnx.org/contents/[email protected]|title=Anatomy and Physiology|publisher=Rice University (OpenStax)|year=2016-02-26|isbn=|location=|pages=}}</ref> |
|||
* ''Interoceptive senses'' are senses that perceive [[#Other internal senses|sensations in internal organs]]. |
|||
=== Receptors === |
|||
Non-human animals may possess senses that are absent in humans, such as [[electroreception]] and detection of [[polarized light]]. |
|||
{{Main|Sensory receptor}} |
|||
Sensory receptors are the cells or structures that detect sensations. [[Stimulus (physiology)|Stimuli]] in the environment activate specialized receptor cells in the [[peripheral nervous system]]. During transduction, physical stimulus is converted into [[action potential]] by receptors and transmitted towards the [[central nervous system]] for processing.<ref name="mcb" /> Different types of stimuli are sensed by different types of [[Sensory neuron|receptor cells]]. Receptor cells can be classified into types on the basis of three different criteria: [[Sensory neuron|cell type]], position, and function. Receptors can be classified structurally on the basis of cell type and their position in relation to stimuli they sense. Receptors can further be classified functionally on the basis of the [[Transduction (physiology)|transduction]] of stimuli, or how the mechanical stimulus, light, or chemical changed the cell [[membrane potential]].<ref name=":0" /> |
|||
==== Structural receptor types ==== |
|||
In [[Buddhist philosophy]], [[Ayatana]] or "sense-base" includes the mind as a sense organ, in addition to the traditional five. This addition to the commonly acknowledged senses may arise from the psychological orientation involved in Buddhist thought and practice. The mind considered by itself is seen as the principal gateway to a different spectrum of phenomena that differ from the physical sense data. This way of viewing the human sense system indicates the importance of internal sources of sensation and perception that complements our experience of the external world. |
|||
===== Location ===== |
|||
==Aristotelian senses== |
|||
One way to classify receptors is based on their location relative to the stimuli. An [[Exteroception|exteroceptor]] is a receptor that is located near a stimulus of the external environment, such as the somatosensory receptors that are located in the skin. An [[Interoception|interoceptor]] is one that interprets stimuli from internal organs and tissues, such as the receptors that sense the increase in blood pressure in the [[aorta]] or [[carotid sinus]].<ref name=":0" /> |
|||
{{see also|Five wits#The "outward" wits}} |
|||
=== |
===== Cell type ===== |
||
The cells that interpret information about the environment can be either (1) a [[neuron]] that has a [[free nerve ending]], with [[Dendrite|dendrites]] embedded in tissue that would receive a sensation; (2) a neuron that has an encapsulated ending in which the sensory nerve endings are encapsulated in [[connective tissue]] that enhances their sensitivity; or (3) a specialized [[Sensory neuron|receptor cell]], which has distinct structural components that interpret a specific type of stimulus. The [[Nociceptor|pain]] and [[Thermoreceptor|temperature receptors]] in the dermis of the skin are examples of neurons that have free nerve endings (1). Also located in the dermis of the skin are [[Pacinian corpuscle|lamellated corpuscles]], neurons with encapsulated nerve endings that respond to pressure and touch (2). The cells in the retina that respond to light stimuli are an example of a specialized receptor (3), a [[Photoreceptor cell|photoreceptor]].<ref name=":0" /> |
|||
A [[transmembrane protein]] receptor is a protein in the [[cell membrane]] that mediates a physiological change in a neuron, most often through the opening of [[Ion channel|ion channels]] or changes in the [[cell signaling]] processes. Transmembrane receptors are activated by chemicals called [[Ligand|ligands]]. For example, a molecule in food can serve as a ligand for taste receptors. Other transmembrane proteins, which are not accurately called receptors, are sensitive to mechanical or thermal changes. Physical changes in these proteins increase ion flow across the membrane, and can generate an [[action potential]] or a [[graded potential]] in the [[Sensory neuron|sensory neurons]].<ref name=":0" /> |
|||
[[Image: Pietro Paolini - Allegory of the Five Senses - Walters 372768.jpg|left|thumb|In this painting by [[Pietro Paolini]], each individual represents one of the five senses.<ref>{{cite web |publisher= [[The Walters Art Museum]] |
|||
|url= http://art.thewalters.org/detail/15244 |
|||
|title=Allegory of the Five Senses }}</ref>]] |
|||
[[Visual perception|Sight]] or vision (adjectival form: visual/optical) is the capability of the eye(s) to focus and detect images of visible [[light]] on [[Photoreceptor cell|photoreceptors]] in the [[retina]] of each eye that generates electrical [[Action potential|nerve impulses]] for varying colors, hues, and brightness. There are two types of photoreceptors: [[Rod cell|rods]] and [[Cone cell|cones]]. Rods are very sensitive to light but do not distinguish colors. Cones distinguish colors but are less sensitive to dim light. There is some disagreement as to whether this constitutes one, two or three senses. Neuroanatomists generally regard it as two senses, given that different receptors are responsible for the perception of color and brightness. Some argue{{Citation needed|date=December 2007}} that [[stereopsis]], the perception of depth using both eyes, also constitutes a sense, but it is generally regarded as a cognitive (that is, post-sensory) function of the [[visual cortex]] of the brain where patterns and objects in [[Real image|images]] are recognized and interpreted based on previously learned information. This is called visual memory. |
|||
==== Functional receptor types ==== |
|||
The inability to see is called [[blindness]]. Blindness may result from damage to the eyeball, especially to the retina, damage to the optic nerve that connects each eye to the brain, and/or from [[stroke]] ([[infarct]]s in the brain). Temporary or permanent blindness can be caused by poisons or medications. |
|||
A third classification of receptors is by how the receptor [[Transduction (physiology)|transduces]] stimuli into [[membrane potential]] changes. Stimuli are of three general types. Some stimuli are ions and [[Macromolecule|macromolecules]] that affect transmembrane receptor proteins when these chemicals diffuse across the cell membrane. Some stimuli are physical variations in the environment that affect receptor cell membrane potentials. Other stimuli include the electromagnetic radiation from visible light. For humans, the only electromagnetic energy that is perceived by our eyes is visible light. Some other organisms have receptors that humans lack, such as the heat sensors of snakes, the ultraviolet light sensors of bees, or magnetic receptors in migratory birds.<ref name=":0" /> |
|||
Receptor cells can be further categorized on the basis of the type of stimuli they transduce. The different types of functional receptor cell types are [[mechanoreceptor]]<nowiki/>s, [[Photoreceptor cell|photoreceptors]], [[Chemoreceptor|chemoreceptors]] ([[osmoreceptor]]), [[Thermoreceptor|thermoreceptors]], and [[Nociceptor|nociceptors]]. Physical stimuli, such as pressure and vibration, as well as the sensation of sound and body position (balance), are interpreted through a mechanoreceptor. Photoreceptors convert light (visible [[electromagnetic radiation]]) into signals. Chemical stimuli can be interpreted by a chemoreceptor that interprets chemical stimuli, such as an object’s taste or smell, while osmoreceptors respond to a chemical solute concentrations of body fluids. Nociception (pain) interprets the presence of tissue damage, from sensory information from mechano-, chemo-, and thermoreceptors.<ref>{{Cite book|url=https://www.worldcat.org/oclc/27216558|title=Principles of neural science|date=1991|publisher=Appleton & Lange|others=Kandel, Eric R., Schwartz, James H. (James Harris), 1932-2006., Jessell, Thomas M.|isbn=0-8385-8034-3|edition=3rd ed|location=Norwalk, Conn.|oclc=27216558}}</ref> Another physical stimulus that has its own type of receptor is temperature, which is sensed through a [[thermoreceptor]] that is either sensitive to temperatures above (heat) or below (cold) normal body temperature.<ref name=":0" /> |
|||
People who are blind from degradation or damage to the visual cortex, but still have functional eyes, are actually capable of some level of vision and reaction to visual stimuli but not a conscious perception; this is known as [[blindsight]]. People with blindsight are usually not aware that they are reacting to visual sources, and instead just unconsciously adapt their behavior to the stimulus. |
|||
=== Thresholds === |
|||
On February 14, 2013 researchers developed a [[neural implant]] that gives [[rat]]s the ability to sense [[infrared]] light which for the first time provides [[living creatures]] with new abilities, instead of simply replacing or augmenting existing abilities.<ref>{{cite journal|url=https://www.wired.co.uk/news/archive/2013-02/14/implant-gives-rats-sixth-sense-for-infrared-light|title=Implant gives rats sixth sense for infrared light|journal=Wired UK|date=14 February 2013|access-date=14 February 2013}}</ref> |
|||
=== |
==== Absolute threshold ==== |
||
{{Main|Absolute threshold}} |
|||
Each [[Sensory organ|sense organ]] (eyes or nose, for instance) requires a minimal amount of stimulation in order to detect a stimulus. This minimum amount of stimulus is called the absolute threshold.<ref name="pri" /> The absolute threshold is defined as the minimum amount of stimulation necessary for the detection of a stimulus 50% of the time.<ref name="wol">{{cite book|last1=Wolfe|first1=Jeremy|title=Sensation & perception|last2=Kluender|first2=Keith|last3=Levi|first3=Dennis|publisher=Sinauer Associates|isbn=978-0-87893-572-7|edition=3rd|page=7}}</ref> Absolute threshold is measured by using a method called [[signal detection]]. This process involves presenting stimuli of varying intensities to a subject in order to determine the level at which the subject can reliably detect stimulation in a given sense.<ref name="pri" /> |
|||
==== Differential threshold ==== |
|||
[[Hearing (sense)|Hearing]] or audition (adjectival form: auditory) is the sense of [[sound]] perception. Hearing is all about vibration. Mechanoreceptors turn motion into electrical nerve pulses, which are located in the inner ear. Since sound is vibration, propagating through a medium such as air, the detection of these vibrations, that is the sense of the hearing, is a mechanical sense because these vibrations are mechanically conducted from the eardrum through a series of tiny bones to hair-like fibers in the [[inner ear]], which detect mechanical motion of the fibers within a range of about 20 to 20,000 [[hertz]],<ref>{{cite web|url=http://hypertextbook.com/facts/2003/ChrisDAmbrose.shtml |title=Frequency Range of Human Hearing, Physics Factbook by Glenn Elert (ed) |publisher=Hypertextbook.com |date= |access-date=2014-04-05}}</ref> with substantial variation between individuals. Hearing at high frequencies declines with an increase in age. Inability to hear is called [[deafness]] or hearing impairment. Sound can also be detected as [[Seismic communication|vibrations conducted through the body]] by tactition. Lower frequencies that can be heard are detected this way. Some deaf people are able to determine the direction and location of vibrations picked up through the feet.<ref>{{cite web|url=http://www.vicdeaf.com.au/files/editor_upload/File/Information%20Sheets/Deaf%20Culture%20and%20Communication%20-%20A%20Basic%20Guide.pdf|title=Deaf Culture and Communication: A Basic Guide|work=Victorian Deaf Society|year=2010}}</ref> |
|||
{{Main|Differential threshold}} |
|||
Differential threshold or just noticeable difference (JDS) is the smallest detectable difference between two stimuli, or the smallest difference in stimuli that can be judged to be different from each other.<ref name="wol" /> [[Weber's Law]] is an empirical law that states that the difference threshold is a constant fraction of the comparison stimulus.<ref name="wol" /> According to Weber's Law, bigger stimuli require larger differences to be noticed.<ref name="pri" /> |
|||
[[File:Power_exponents_and_Steven's_Power_Law.svg|thumb|Human power exponents and Steven's Power Law]] |
|||
[[Psychophysics#Magnitude estimation|Magnitude estimation]] is a [[Psychophysics|psychophysical]] method in which subjects assign perceived values of given stimuli. The relationship between stimulus intensity and perceptive intensity is described by [[Steven's power law]].<ref name="wol" /> |
|||
=== Signal detection theory === |
|||
===Taste=== |
|||
{{Main|Signal detection theory}} |
|||
Signal detection theory quantifies the experience of the subject to the presentation of a stimulus in the presence of [[Noise (signal processing)|noise]]. There is internal noise and there is external noise when it comes to signal detection. The internal noise originates from static in the nervous system. For example, an individual with closed eyes in a dark room still sees something - a blotchy pattern of grey with intermittent brighter flashes -, this is internal noise. External noise is the result of noise in the environment that can interfere with the detection of the stimulus of interest. Noise is only a problem if the magnitude of the noise is large enough to interfere with signal collection. The [[nervous system]] calculates a criterion, or an internal threshold, for the detection of a signal in the presence of noise. If a signal is judged to be above the criterion, thus the signal is differentiated from the noise, the signal is sensed and perceived. Errors in signal detection can potentially lead to [[false positives and false negatives]]. The sensory criterion might be shifted based on the importance of the detecting the signal. Shifting of the criterion may influence the likelihood of false positives and false negatives.<ref name="wol" /> |
|||
=== Private perceptive experience === |
|||
[[Taste]] or gustation (adjectival form: gustatory) is one of the traditional five senses. It refers to the capability to detect the taste of substances such as food, certain minerals, and poisons, etc. The sense of taste is often confused with the "sense" of flavor, which is a combination of taste and smell perception. [[File:Philippe Mercier - The Sense of Taste - Google Art Project.jpg|thumb|Philippe Mercier - The Sense of Taste - Google Art Project]] Flavor depends on odor, texture, and temperature as well as on taste. Humans receive tastes through sensory organs called taste buds, or gustatory calyculi, concentrated on the upper surface of the tongue. There are five [[basic taste]]s: [[sweet]], [[Bitter (taste)#Bitterness|bitter]], [[sour]], [[Saltiness|salty]] and [[umami]]. Other tastes such as calcium<ref name="Tordoff 2008">{{cite journal | vauthors = Tordoff MG | title = Gene discovery and the genetic basis of calcium consumption | journal = Physiology & Behavior | volume = 94 | issue = 5 | pages = 649–59 | date = August 2008 | pmid = 18499198 | pmc = 2574908 | doi = 10.1016/j.physbeh.2008.04.004 }}</ref><ref name="Sciencedaily 2008">{{cite web |url=https://www.sciencedaily.com/releases/2008/08/080820163008.htm |title=That Tastes ... Sweet? Sour? No, It's Definitely Calcium! |work=Sciencedaily}}</ref> and [[free fatty acids]]<ref name="Mattes 2009">{{cite journal | vauthors = Mattes RD | title = Is there a fatty acid taste? | journal = Annual Review of Nutrition | volume = 29 | issue = | pages = 305–27 | year = 2009 | pmid = 19400700 | pmc = 2843518 | doi = 10.1146/annurev-nutr-080508-141108 }}</ref> may also be basic tastes but have yet to receive widespread acceptance. The inability to taste is called [[ageusia]]. |
|||
{{Main|Qualia}} |
|||
Subjective visual and auditory experiences appear to be similar across humans subjects. The same cannot be said about taste. For example, there is a molecule called [[propylthiouracil]] (PROP) that some humans experience as bitter, some as almost tasteless, while others experience it as somewhere between tasteless and bitter. There is a genetic basis for this difference between perception given the same sensory stimulus. This subjective difference in taste perception has implications for individuals' food preferences, and consequently, health.<ref name="wol" /> |
|||
=== |
=== Sensory adaptation === |
||
{{Main|Sensory adaptation}} |
|||
When a stimulus is constant and unchanging, perceptual sensory adaptation occurs. During this process, the subject becomes less sensitive to the stimulus.<ref name="pri" /> |
|||
=== Fourier analysis === |
|||
[[Olfaction|Smell]] or olfaction (adjectival form: olfactory) is the other "chemical" sense. Unlike taste, there are hundreds of olfactory receptors (388 according to one source<ref name=Moonwalk |
|||
{{Main|Fourier analysis}} |
|||
>{{cite web |url=http://sandwalk.blogspot.co.uk/2007/01/sense-of-smell-olfactory-receptors.html |title=A Sense of Smell: Olfactory Receptors |work=Sandwalk |access-date=|date=2007-01-09 }}</ref>), each binding to a particular molecular feature. Odor molecules possess a variety of features and, thus, excite specific receptors more or less strongly. This combination of excitatory signals from different receptors makes up what we perceive as the molecule's smell. [[File:The sense of smell Philippe Mercier.jpg|thumb|The sense of smell Bequest of [[Mrs E.G. Elgar]], 1945 [[Museum of New Zealand Te Papa Tongarewa]].]] In the brain, olfaction is processed by the [[olfactory system]]. [[Olfactory receptor neuron]]s in the [[Human nose|nose]] differ from most other neurons in that they die and regenerate on a regular basis. The inability to smell is called [[anosmia]]. Some neurons in the nose are specialized to detect [[pheromone]]s.<ref name="LiveScience">{{cite web |url=http://www.livescience.com/2737-surprising-impact-taste-smell.html |title=The Surprising Impact of Taste and Smell |work=LiveScience }}</ref> |
|||
Biological auditory (hearing), vestibular and spatial, and visual systems (vision) appear to break down real-world complex stimuli into [[sine wave]] components, through the mathematical process called Fourier analysis. Many neurons have a strong preference for certain sine [[frequency]] components in contrast to others. The way how simpler sounds and images are [[Code|encoded]] during sensation can provide insight into how perception of real-world objects happen.<ref name="wol" /> |
|||
=== Sensory neuroscience and the biology of perception === |
|||
===Touch=== |
|||
{{Main|Sensory neuroscience}} |
|||
Perception occurs when [[Nerve|nerves]] that lead from the [[Sensory nervous system|sensory organs]] (e.g. eye) to the brain are stimulated, even if that stimulation is unrelated to the target signal of the sensory organ. For example, in the case of the eye, it does not matter whether light or something else stimulates the optic nerve, that stimulation will results in visual perception, even if there was no visual stimulus to begin with. (To prove this point to yourself (and if you are a human), close your eyes (preferably in a dark room) and press gently on the outside corner of one eye through the eyelid. You will see a visual spot toward the inside of your visual field, near your nose.)<ref name="wol" /> |
|||
==== Sensory nervous system ==== |
|||
[[Somatosensory system|Touch]] or somatosensation (adjectival form: somatic), also called [[tactition]] (adjectival form: tactile) or [[Mechanoreceptor|mechanoreception]], is a perception resulting from activation of neural [[Mechanoreceptor|receptors]], generally in the [[skin]] including [[hair follicle]]s, but also in the [[tongue]], [[throat]], and [[Mucous membrane|mucosa]]. A variety of [[pressure]] receptors respond to variations in pressure (firm, brushing, sustained, etc.). The touch sense of [[itch]]ing caused by insect bites or allergies involves special itch-specific neurons in the skin and spinal cord.<ref name="Sun Zhao 2009">{{cite journal | vauthors = Sun YG, Zhao ZQ, Meng XL, Yin J, Liu XY, Chen ZF | title = Cellular basis of itch sensation | journal = Science | volume = 325 | issue = 5947 | pages = 1531–4 | date = September 2009 | pmid = 19661382 | pmc = 2786498 | doi = 10.1126/science.1174868 | bibcode = 2009Sci...325.1531S }}</ref> The loss or impairment of the ability to feel anything touched is called tactile [[anesthesia]]. [[Paresthesia]] is a sensation of tingling, pricking, or [[numbness]] of the skin that may result from nerve damage and may be permanent or temporary. |
|||
{{Main|Sensory nervous system}} |
|||
All stimuli received by the [[Sensory neuron|receptors]] are [[Transduction (physiology)|transduced]] to an [[action potential]], which is carried along one or more afferent [[neuron]]<nowiki/>s towards a specific area ([[Cortex (anatomy)|cortex]]) of the [[brain]]. Just as different nerves are dedicated to sensory and motors tasks, different areas of the brain (cortices) are similarly dedicated to different [[Sensory cortex|sensory]] and [[Perception|perceptual]] tasks. More complex processing is accomplished across primary cortical regions that spread beyond the primary cortices. Every nerve, [[Sensory nerve|sensory]] or [[Motor nerve|motor]], has its own signal transmission speed. For example, nerves in the frog's legs have a 90 ft/s (99 km/h) signal transmission speed, while sensory nerves in humans, transmit sensory information at speeds between 165 ft/s (181 km/h) and 330 ft/s (362 km/h).<ref name="wol" /> |
|||
{| class="wikitable" |
|||
|+The [[human]] external sensory and perceptual system<ref name="wol" /><ref name=":0" /> |
|||
![[Stimulus (physiology)|Physical stimulus]] |
|||
!Sensory organ |
|||
![[Sensory receptor]] |
|||
![[Sensory system]] |
|||
![[Cranial nerves|Cranial nerve(s)]] |
|||
![[Cerebral cortex]] |
|||
!Primary associated [[Perception|perception(s]]) |
|||
!Name |
|||
|- |
|||
|[[Light]] |
|||
|[[Human eye|Eyes]] |
|||
|[[Photoreceptor cell|Photoreceptor]] |
|||
|[[Visual system]] |
|||
|[[Optic (II) nerve|Optic (II)]] |
|||
|[[Visual cortex]] |
|||
|[[Visual perception]] |
|||
|[[Vision]] |
|||
|- |
|||
|[[Sound]] |
|||
|[[Ear|Ears]] |
|||
|[[Mechanoreceptor]] |
|||
|[[Auditory system]] |
|||
|[[Vestibulocochlear nerve|Vestibulocochlear (VIII)]] |
|||
|[[Auditory cortex]] |
|||
|[[Auditory perception]] |
|||
|[[Hearing|Hearing (audition)]] |
|||
|- |
|||
|[[Gravity]] and [[acceleration]] |
|||
|[[Inner ear]] |
|||
|Mechanoreceptor |
|||
|[[Vestibular system]] |
|||
|Vestibulocochlear (VIII) |
|||
|[[Vestibular cortex]] |
|||
|[[Equilibrioception]] |
|||
|[[Balance (ability)|Balance (equilibrium)]] |
|||
|- |
|||
|[[Chemical substance]] |
|||
|[[Human nose|Nose]] |
|||
|[[Chemoreceptor]] |
|||
|[[Olfactory system]] |
|||
|[[Olfactory nerve|Olfactory (I)]] |
|||
|[[Olfactory cortex]] |
|||
|[[Olfactory perception]], Gustatory perception (taste or flavor)<ref>Small DM, Green BG. A Proposed Model of a Flavor Modality. In: Murray MM, Wallace MT, editors. The Neural Bases of Multisensory Processes. Boca Raton (FL): CRC Press/Taylor & Francis; 2012. Chapter 36. Available from: https://www.ncbi.nlm.nih.gov/books/NBK92876/</ref> |
|||
|[[Olfaction|Smell (olfaction)]] |
|||
|- |
|||
|Chemical substance |
|||
|[[Human mouth|Mouth]] |
|||
|Chemoreceptor |
|||
|[[Gustatory system]] |
|||
|[[Facial nerve|Facial (VII)]], [[Glossopharyngeal nerve|Glossopharyngeal (IX)]] |
|||
|[[Gustatory cortex]] |
|||
|[[Flavor|Gustatory perception (taste or flavor)]] |
|||
|[[Taste|Taste (gustation)]] |
|||
|- |
|||
|[[Position (geometry)|Position]], [[motion]], [[temperature]] |
|||
|[[Human skin|Skin]] |
|||
|Mechanoreceptor,[[thermoreceptor]] |
|||
|[[Somatosensory system]] |
|||
|[[Trigeminal nerve|Trigeminal (V)]], Glossopharyngeal (IX) ) + [[Spinal nerve]]<nowiki/>s |
|||
|[[Somatosensory cortex]] |
|||
|[[Tactile perception]] ([[mechanoreception]], [[thermoception]]) |
|||
|[[Touch|Touch (tactition)]] |
|||
|} |
|||
== |
=== Multimodal perception === |
||
{{Main|Multimodal integration}} |
|||
Perceptual experience is often multimodal. Multimodality integrates different senses into one unified perceptual experience. Information from one sense has the potential to influence how information from another is perceived.<ref name="pri" /> Multimodal perception is qualitatively different from unimodal perception. There has been a growing body of evidence since the mid-90’s on the neural correlates of multimodal perception.<ref name="cal">Calvert, G.A., Hansen, P.C., Iversen, S.D. and Brammer, M.J., 2001. Detection of audio-visual integration sites in humans by application of electrophysiological criteria to the BOLD effect. Neuroimage, 14(2), pp.427-438.</ref> |
|||
=== |
=== Philosophy === |
||
{{Main| |
{{Main|Philosophy of perception}} |
||
Historical inquiries into the underlying mechanisms of sensation and perception have lead early researchers to subscribe to various philosophical interpretations of perception and the [[Philosophy of mind|mind]], including [[panpsychism]], [[Mind–body dualism|dualism]], and [[materialism]]. The majority of modern scientists who study sensation and perception takes on a materialistic view of the mind.<ref name="wol" /> |
|||
== Human sensation == |
|||
[[Balance (ability)|Balance]], [[equilibrioception]], or vestibular sense is the sense that allows an organism to sense body movement, direction, and acceleration, and to attain and maintain postural equilibrium and balance. The organ of equilibrioception is the vestibular labyrinthine system found in both of the [[inner ear]]s. In technical terms, this organ is responsible for two senses of [[angular momentum]] acceleration and [[linear acceleration]] (which also senses [[gravity]]), but they are known together as equilibrioception. |
|||
=== General === |
|||
The [[vestibular nerve]] conducts information from sensory receptors in three [[Osseous ampullae|ampulla]] that sense motion of fluid in three [[semicircular canal]]s caused by three-dimensional rotation of the head. The vestibular nerve also conducts information from the [[utricle (ear)|utricle]] and the [[saccule]], which contain hair-like sensory receptors that bend under the weight of [[otolith]]s (which are small crystals of [[calcium carbonate]]) that provide the inertia needed to detect head rotation, linear acceleration, and the direction of gravitational force. |
|||
=== |
==== Absolute Threshold ==== |
||
Some examples of [[human]] [[Absolute threshold|absolute thresholds]] for the six [[Sensory nervous system|external senses]].<ref name="gal">{{cite journal|last1=Galanter|first1=E.|date=1962|title=Direct measurement of utility and subjective probability|journal=American Journal of Psychology|volume=75|pages=208-220}}</ref> |
|||
{| class="wikitable" |
|||
!Sense |
|||
!Absolute threshold |
|||
|- |
|||
|Vision |
|||
|Stars at night; Candlelight 48 km (30 mi) away on a dark and clear night |
|||
|- |
|||
|Hearing |
|||
|Ticking of a watch 6 m (20 ft) away, in an otherwise silent environment |
|||
|- |
|||
|Vestibular |
|||
|Tilt of less 30 seconds (0.0083 degrees) on a clocks face |
|||
|- |
|||
|Touch |
|||
|A wing of fly falling on the cheek from a height of 7.6 cm (3 inches) |
|||
|- |
|||
|Taste |
|||
|A teaspoon of sugar in 7.5 liters (2 gallons) of water |
|||
|- |
|||
|Smell |
|||
|A drop of perfume in a volume of the size of three rooms |
|||
|} |
|||
==== Multimodal perception ==== |
|||
[[Thermoception]] is the sense of heat and the absence of heat (cold) by the [[skin]] and internal skin passages, or, rather, the [[heat flux]] (the rate of [[heat flow]]) in these areas. There are specialized receptors for cold (declining temperature) and for heat (increasing temperature). The cold receptors play an important part in the animal's sense of smell, telling wind direction. The heat receptors are sensitive to infrared radiation and can occur in specialized organs, for instance in [[pit vipers]]. The [[Thermoreceptor|thermoceptors]] in the skin are quite different from the [[homeostasis|homeostatic]] thermoceptors in the brain ([[POAH|hypothalamus]]), which provide feedback on internal body temperature. |
|||
[[Human|Humans]] respond more strongly to [[Multisensory integration|multimodal stimuli]] compared to the sum of each single modality together, an effect called the ''superadditive effect of multisensory integration''.<ref name="pri" /> Neurons that respond to both visual and auditory stimuli have been identified in the [[superior temporal sulcus]].<ref name="cal" /> Additionally, multimodal “what” and “where” pathways have been proposed for auditory and tactile stimuli.<ref name="ren">Renier, L.A., Anurova, I., De Volder, A.G., Carlson, S., VanMeter, J. and Rauschecker, J.P., 2009. Multisensory integration of sounds and vibrotactile stimuli in processing streams for “what” and “where”. Journal of Neuroscience, 29(35), pp.10950-10960.</ref> |
|||
=== |
=== External === |
||
External receptors that respond to stimuli from outside the body are called [[Extoreceptor|extoreceptors]].<ref>{{Cite book|last=Campbell, Neil A. (1946-2004).|url=http://worldcat.org/oclc/904492777|title=Biology|date=cop. 2005|publisher=Pearson|isbn=0-321-26984-5|oclc=904492777}}</ref> Human external sensation is based on the sensory organs of the [[Human eye|eyes]], [[Human ear|ears]], [[Human skin|skin]], [[vestibular system]], [[Human nose|nose]], and [[Human mouth|mouth]], which contribute, respectively, to the sensory [[Perception|perceptions]] of [[Visual perception|vision]], [[hearing]], [[touch]], [[Equilibrioception|spatial orientation]], [[Olfaction|smell]], and [[Flavor|taste]]. Smell and taste are both responsible for identifying molecules and thus both are types of [[chemoreceptor]]<nowiki/>s. Both olfaction (smell) and gustation (taste) require the transduction of chemical stimuli into electrical potentials.<ref name="pri" /><ref name="wol" /> |
|||
==== Visual system (vision) ==== |
|||
[[Proprioception]], the kinesthetic sense, provides the [[Parietal lobe|parietal cortex]] of the brain with information on the movement and relative positions of the parts of the body. Neurologists test this sense by telling patients to close their eyes and touch their own nose with the tip of a finger. Assuming proper proprioceptive function, at no time will the person lose awareness of where the hand actually is, even though it is not being detected by any of the other senses. Proprioception and touch are related in subtle ways, and their impairment results in surprising and deep deficits in perception and action.<ref name="roblesdelatorre">{{cite web |url=http://www.roblesdelatorre.com/gabriel/GR-IEEE-MM-2006.pdf |title=The Importance of the Sense of Touch in Virtual and Real Environments |work=International Society for Haptics |access-date=}}</ref> |
|||
{{Main|Visual system}} |
|||
The visual system, or sense of sight, is based on the transduction of light stimuli received through the eyes and contributes to [[visual perception]]. The visual system detects [[light]] on [[Photoreceptor cell|photoreceptors]] in the [[retina]] of each eye that generates electrical [[Action potential|nerve impulses]] for the perception of varying colors and brightness. There are two types of photoreceptors: [[Rod cell|rods]] and [[Cone cell|cones]]. Rods are very sensitive to light but do not distinguish colors. Cones distinguish colors but are less sensitive to dim light.<ref name=":0" /> |
|||
At the molecular level, visual stimuli cause changes in the photopigment molecule that lead to changes in membrane potential of the photoreceptor cell. A single unit of light is called a [[photon]], which is described in physics as a packet of energy with properties of both a particle and a wave. The [[energy]] of a photon is represented by its [[wavelength]], with each wavelength of visible light corresponding to a particular [[color]]. Visible light is [[electromagnetic radiation]] with a wavelength between 380 and 720 nm. Wavelengths of electromagnetic radiation longer than 720 nm fall into the [[infrared]] range, whereas wavelengths shorter than 380 nm fall into the [[ultraviolet]] range. Light with a wavelength of 380 nm is [[blue]] whereas light with a wavelength of 720 nm is dark [[red]]. All other colors fall between red and blue at various points along the wavelength scale.<ref name=":0" /> |
|||
===Pain=== |
|||
The three types of cone [[opsin]]<nowiki/>s, being sensitive to different wavelengths of light, provide us with color vision. By comparing the activity of the three different cones, the brain can extract color information from visual stimuli. For example, a bright blue light that has a wavelength of approximately 450 nm would activate the “red” cones minimally, the “green” cones marginally, and the “blue” cones predominantly. The relative activation of the three different cones is calculated by the brain, which perceives the color as blue. However, cones cannot react to low-intensity light, and rods do not sense the color of light. Therefore, our low-light vision is—in essence—in [[grayscale]]. In other words, in a dark room, everything appears as a shade of [[Grey|gray]]. If you think that you can see colors in the dark, it is most likely because your brain knows what color something is and is relying on that memory.<ref name=":0" /> |
|||
[[Nociception]] (physiological [[pain]]) signals nerve-damage or damage to tissue. The three types of pain receptors are cutaneous (skin), somatic (joints and bones), and visceral (body organs). It was previously believed that pain was simply the overloading of pressure receptors, but research in the first half of the 20th century indicated that pain is a distinct phenomenon that intertwines with all of the other senses, including touch. Pain was once considered an entirely subjective experience, but recent studies show that pain is registered in the [[anterior cingulate gyrus]] of the brain.<ref name="Fulbright 2001">{{cite journal | authors = Fulbright RK, Troche CJ, Skudlarski P, Gore JC, Wexler BE | title = Functional MR imaging of regional brain activation associated with the affective experience of pain | journal = AJR. American Journal of Roentgenology | volume = 177 | issue = 5 | pages = 1205–10 | date = November 2001 | pmid = 11641204 | doi = 10.2214/ajr.177.5.1771205 }}</ref> The main function of pain is to attract our [[attention]] to dangers and motivate us to avoid them. For example, humans avoid touching a sharp needle, or hot object, or extending an arm beyond a safe limit because it is dangerous, and thus hurts. Without pain, people could do many dangerous things without being aware of the dangers. |
|||
There is some disagreement as to whether the visual system consists of one, two, or thre submodalities. Neuroanatomists generally regard it as two submodalities, given that different receptors are responsible for the perception of color and brightness. Some argue{{Citation needed|date=December 2007}} that [[stereopsis]], the perception of depth using both eyes, also constitutes a sense, but it is generally regarded as a cognitive (that is, post-sensory) function of the [[visual cortex]] of the brain where patterns and objects in [[Real image|images]] are recognized and interpreted based on previously learned information. This is called visual memory. |
|||
=== Magnetoception === |
|||
[[Magnetoception]] (or magnetoreception) is the ability to detect the direction one is facing based on the Earth's [[magnetic field]]. Directional awareness is most commonly observed in [[bird]]s, which rely on their magnetic sense to navigate during migration.<ref name="Theoretical and Computational Biophysics Group">{{cite web|url=http://www.ks.uiuc.edu/Research/magsense/ms.html|title=The Magnetic Sense of Animals|work=Theoretical and Computational Biophysics Group}}</ref><ref name="Theoretical and Computational Biophysics Group" /><ref name="Baylor College of Medicine">{{cite web|url=http://www.bcm.edu/news/item.cfm?newsID=5575|title=Built-in GPS in birds in tune with Earth's magnetic field|work=Baylor College of Medicine|access-date=}}</ref>{{Dead link | date = May 2019 | fix-attempted = yes }}<ref name="Wu Dickman 2012">{{cite journal | vauthors = Wu LQ, Dickman JD | title = Neural correlates of a magnetic sense | journal = Science | volume = 336 | issue = 6084 | pages = 1054–7 | date = May 2012 | pmid = 22539554 | doi = 10.1126/science.1216567 | bibcode = 2012Sci...336.1054W }}</ref><ref name="Cressey2012">{{cite journal|last1=Cressey|first1=Daniel |name-list-format = vanc |year=2012|title=Pigeons may 'hear' magnetic fields|journal=Nature|doi=10.1038/nature.2012.10540|issn=1744-7933}}</ref> It has also been observed in insects such as [[bee]]s. Cattle make use of magnetoception to align themselves in a north–south direction.<ref name="BBC News - Science/Nature">{{cite news|url=http://news.bbc.co.uk/1/hi/sci/tech/7575459.stm|title=Cattle shown to align north-south|work=BBC News - Science/Nature}}</ref> [[Magnetotactic bacteria]] build miniature magnets inside themselves and use them to determine their orientation relative to the Earth's magnetic field.<ref name="Blakemore 1975">{{cite journal | vauthors = Blakemore R | title = Magnetotactic bacteria | journal = Science | volume = 190 | issue = 4212 | pages = 377–9 | date = October 1975 | pmid = 170679 | doi = 10.1126/science.170679 | bibcode = 1975Sci...190..377B }}</ref><ref name="Urban 2000">{{cite journal | vauthors = Urban JE | title = Adverse effects of microgravity on the magnetotactic bacterium Magnetospirillum magnetotacticum | journal = Acta Astronautica | volume = 47 | issue = 10 | pages = 775–80 | date = November 2000 | pmid = 11543576 | doi = 10.1016/S0094-5765(00)00120-X | bibcode = 2000AcAau..47..775U }}</ref> There has been some recent (tentative) research suggesting that the [[Rhodopsin]] in the human eye, which responds particularly well to blue light, can facilitate magnetoception in humans.<ref>{{cite journal | vauthors = Chae KS, Oh IT, Lee SH, Kim SC | title = Blue light-dependent human magnetoreception in geomagnetic food orientation | journal = PLOS ONE | volume = 14 | issue = 2 | pages = e0211826 | date = 2019-02-14 | pmid = 30763322 | pmc = 6375564 | doi = 10.1371/journal.pone.0211826 }}</ref> |
|||
The inability to see is called [[blindness]]. Blindness may result from damage to the eyeball, especially to the retina, damage to the optic nerve that connects each eye to the brain, and/or from [[stroke]] ([[Infarct|infarcts]] in the brain). Temporary or permanent blindness can be caused by poisons or medications. People who are blind from degradation or damage to the visual cortex, but still have functional eyes, are actually capable of some level of vision and reaction to visual stimuli but not a conscious perception; this is known as [[blindsight]]. People with blindsight are usually not aware that they are reacting to visual sources, and instead just unconsciously adapt their behavior to the stimulus. |
|||
===Sexual stimulation=== |
|||
{{Main|Sexual stimulation}} |
|||
On February 14, 2013 researchers developed a [[neural implant]] that gives [[Rat|rats]] the ability to sense [[infrared]] light which for the first time provides [[living creatures]] with new abilities, instead of simply replacing or augmenting existing abilities.<ref>{{cite journal|date=14 February 2013|title=Implant gives rats sixth sense for infrared light|url=https://www.wired.co.uk/news/archive/2013-02/14/implant-gives-rats-sixth-sense-for-infrared-light|journal=Wired UK|access-date=14 February 2013}}</ref> |
|||
[[Sexual stimulation]] is any [[stimulation|stimulus]] (including bodily contact) that leads to, enhances and maintains [[sexual arousal]], and may lead to [[orgasm]]. Distinct from the general sense of touch, sexual stimulation is strongly tied to hormonal activity and chemical triggers in the body. Although sexual arousal may arise without [[physical stimulation]], achieving orgasm usually requires physical sexual stimulation (stimulation of the Krause-Finger [[Bulboid corpuscle|corpuscles]]<ref>{{Cite news|url=https://abdominalkey.com/sensory-corpuscles/|title=Sensory Corpuscles| vauthors = Themes UF |date=2017-03-29|work=Abdominal Key|access-date=2018-07-13}}</ref> found in erogenous zones of the body). |
|||
==== Auditory system (hearing) ==== |
|||
===Other internal senses{{anchor|Interoception}}=== |
|||
{{Main| |
{{Main|Auditory system}} |
||
Hearing, or audition, is the transduction of [[sound waves]] into a neural signal that is made possible by the structures of the [[ear]]. The large, fleshy structure on the lateral aspect of the head is known as the [[Auricle (anatomy)|auricle]]. At the end of the [[auditory canal]] is the tympanic membrane, or [[Eardrum|ear drum]], which vibrates after it is struck by sound waves. The auricle, ear canal, and tympanic membrane are often referred to as the [[external ear]]. The [[middle ear]] consists of a space spanned by three small bones called the [[ossicles]]. The three ossicles are the [[malleus]], [[incus]], and [[stapes]], which are Latin names that roughly translate to hammer, anvil, and stirrup. The malleus is attached to the tympanic membrane and articulates with the incus. The incus, in turn, articulates with the stapes. The stapes is then attached to the [[inner ear]], where the sound waves will be [[Transduction (physiology)|transduced]] into a neural signal. The middle ear is connected to the [[pharynx]] through the [[Eustachian tube]], which helps equilibrate air pressure across the tympanic membrane. The tube is normally closed but will pop open when the muscles of the pharynx contract during [[swallowing]] or [[Yawn|yawning]].<ref name=":0" /> |
|||
An internal sense also known as [[interoception]]<ref name="Craig 2003">{{cite journal | vauthors = Craig AD | title = Interoception: the sense of the physiological condition of the body | journal = Current Opinion in Neurobiology | volume = 13 | issue = 4 | pages = 500–5 | date = August 2003 | pmid = 12965300 | doi = 10.1016/S0959-4388(03)00090-4 }}</ref> is "any sense that is normally stimulated from within the body".<ref name="Dunn Galton 2010">{{cite journal | vauthors = Dunn BD, Galton HC, Morgan R, Evans D, Oliver C, Meyer M, Cusack R, Lawrence AD, Dalgleish T | title = Listening to your heart. How interoception shapes emotion experience and intuitive decision making | journal = Psychological Science | volume = 21 | issue = 12 | pages = 1835–44 | date = December 2010 | pmid = 21106893 | doi = 10.1177/0956797610389191 | url = https://semanticscholar.org/paper/7b2d96919d1befe4fb4ced5ba024339188d27f1c }}</ref> These involve numerous sensory receptors in internal organs. Interoception is thought to be atypical in clinical conditions such as [[alexithymia]].<ref>{{cite journal | vauthors = Shah P, Hall R, Catmur C, Bird G | title = Alexithymia, not autism, is associated with impaired interoception | journal = Cortex; A Journal Devoted to the Study of the Nervous System and Behavior | volume = 81 | pages = 215–20 | date = August 2016 | pmid = 27253723 | pmc = 4962768 | doi = 10.1016/j.cortex.2016.03.021 }}</ref> |
|||
Some examples of specific receptors are: |
|||
* [[Hunger (motivational state)|Hunger]] is a sensation that is governed by a set of brain structures (e.g., the [[hypothalamus]]) that are responsible for [[energy homeostasis]].<ref name="Hunger - CNS energy homeostasis">{{cite journal | vauthors = Farr OM, Li CS, Mantzoros CS | title = Central nervous system regulation of eating: Insights from human brain imaging | journal = Metabolism | volume = 65 | issue = 5 | pages = 699–713 | date = May 2016 | pmid = 27085777 | pmc = 4834455 | doi = 10.1016/j.metabol.2016.02.002 }}</ref> |
|||
* [[Pulmonary stretch receptors]] are found in the lungs and control the [[respiratory rate]]. |
|||
* [[Peripheral chemoreceptors]] in the brain monitor the carbon dioxide and oxygen levels in the brain to give a feeling of [[suffocation]] if carbon dioxide levels get too high.<ref name="HowStuffWorks How Your Lungs Work">{{cite web |url=http://science.howstuffworks.com/life/human-biology/lung3.htm |title=How Your Lungs Work |work=HowStuffWorks |date=2000-10-06 }}</ref> |
|||
* The [[chemoreceptor trigger zone]] is an area of the [[medulla oblongata|medulla]] in the brain that receives inputs from [[blood]]-borne [[drug]]s or [[hormone]]s, and communicates with the [[vomiting center]]. |
|||
* Chemoreceptors in the circulatory system also measure salt levels and prompt thirst if they get too high; they can also respond to high [[blood sugar]] levels in diabetics. |
|||
* [[Cutaneous receptor]]s in the skin not only respond to touch, pressure, temperature and vibration, but also respond to vasodilation in the skin such as [[blushing]]. |
|||
* Stretch receptors in the [[gastrointestinal tract]] sense gas distension that may result in colic pain. |
|||
* Stimulation of sensory receptors in the [[esophagus]] result in sensations felt in the throat when [[swallowing]], [[vomiting]], or during [[acid reflux]]. |
|||
* Sensory receptors in [[pharynx]] mucosa, similar to touch receptors in the skin, sense foreign objects such as mucous and food that may result in a [[gag reflex]] and corresponding gagging sensation. |
|||
* Stimulation of sensory receptors in the [[urinary bladder]] and [[rectum]] may result in sensations of fullness. |
|||
* Stimulation of stretch sensors that sense dilation of various blood vessels may result in pain, for example headache caused by vasodilation of brain arteries. |
|||
* Cardioception refers to the perception of the activity of the heart.<ref>{{cite journal | vauthors = Garfinkel SN, Seth AK, Barrett AB, Suzuki K, Critchley HD | title = Knowing your own heart: distinguishing interoceptive accuracy from interoceptive awareness | journal = Biological Psychology | volume = 104 | pages = 65–74 | date = January 2015 | pmid = 25451381 | doi = 10.1016/j.biopsycho.2014.11.004 }}</ref><ref>{{cite journal | vauthors = Schandry R | title = Heart beat perception and emotional experience | journal = Psychophysiology | volume = 18 | issue = 4 | pages = 483–8 | date = July 1981 | pmid = 7267933 | doi = 10.1111/j.1469-8986.1981.tb02486.x }}</ref><ref>{{cite journal | vauthors = Kleckner IR, Wormwood JB, Simmons WK, Barrett LF, Quigley KS | title = Methodological recommendations for a heartbeat detection-based measure of interoceptive sensitivity | journal = Psychophysiology | volume = 52 | issue = 11 | pages = 1432–40 | date = November 2015 | pmid = 26265009 | pmc = 4821012 | doi = 10.1111/psyp.12503 }}</ref><ref>{{cite journal | vauthors = Whitehead WE, Drescher VM, Heiman P, Blackwell B | title = Relation of heart rate control to heartbeat perception | journal = Biofeedback and Self-Regulation | volume = 2 | issue = 4 | pages = 317–92 | date = December 1977 | pmid = 612350 | doi = 10.1007/BF00998623 }}</ref> |
|||
* [[Opsin]]s and [[direct DNA damage]] in [[melanocyte]]s and [[keratinocyte]]s can sense [[ultraviolet]] radiation, which plays a role in pigmentation and [[sunburn]]. |
|||
*[[Baroreceptor|Baroreceptors]] relay blood pressure information to the brain and maintain proper homeostatic blood pressure. |
|||
Mechanoreceptors turn motion into electrical nerve pulses, which are located in the inner ear. Since sound is vibration, propagating through a medium such as air, the detection of these vibrations, that is the sense of the hearing, is a mechanical sense because these vibrations are mechanically conducted from the eardrum through a series of tiny bones to hair-like fibers in the [[inner ear]], which detect mechanical motion of the fibers within a range of about 20 to 20,000 [[hertz]],<ref>{{cite web|url=http://hypertextbook.com/facts/2003/ChrisDAmbrose.shtml|title=Frequency Range of Human Hearing, Physics Factbook by Glenn Elert (ed)|date=|publisher=Hypertextbook.com|access-date=2014-04-05}}</ref> with substantial variation between individuals. Hearing at high frequencies declines with an increase in age. Inability to hear is called [[deafness]] or hearing impairment. Sound can also be detected as [[Seismic communication|vibrations conducted through the body]] by tactition. Lower frequencies that can be heard are detected this way. Some deaf people are able to determine the direction and location of vibrations picked up through the feet.<ref>{{cite web|url=http://www.vicdeaf.com.au/files/editor_upload/File/Information%20Sheets/Deaf%20Culture%20and%20Communication%20-%20A%20Basic%20Guide.pdf|title=Deaf Culture and Communication: A Basic Guide|year=2010|work=Victorian Deaf Society}}</ref> |
|||
==Perception not based on a specific sensory organ== |
|||
==== Somatosensory system (touch) ==== |
|||
===Time=== |
|||
{{Main|Somatosensory system}} |
|||
{{main | time perception}} |
|||
Somatosensation is considered a general sense, as opposed to the special senses discussed in this section. Somatosensation is the group of sensory modalities that are associated with touch and interoception. The modalities of sometosensation include [[pressure]], [[vibration]], light touch, [[Tickling|tickle]], [[itch]], [[temperature]], [[pain]], [[kinesthesia]].<ref name=":0" /> [[Somatosensation]], also called [[tactition]] (adjectival form: tactile) is a perception resulting from activation of neural [[Mechanoreceptor|receptors]], generally in the [[skin]] including [[Hair follicle|hair follicles]], but also in the [[tongue]], [[throat]], and [[Mucous membrane|mucosa]]. A variety of [[pressure]] receptors respond to variations in pressure (firm, brushing, sustained, etc.). The touch sense of [[Itch|itching]] caused by insect bites or allergies involves special itch-specific neurons in the skin and spinal cord.<ref name="Sun Zhao 2009">{{cite journal|vauthors=Sun YG, Zhao ZQ, Meng XL, Yin J, Liu XY, Chen ZF|date=September 2009|title=Cellular basis of itch sensation|journal=Science|volume=325|issue=5947|pages=1531–4|bibcode=2009Sci...325.1531S|doi=10.1126/science.1174868|pmc=2786498|pmid=19661382}}</ref> The loss or impairment of the ability to feel anything touched is called tactile [[anesthesia]]. [[Paresthesia]] is a sensation of tingling, pricking, or [[numbness]] of the skin that may result from nerve damage and may be permanent or temporary. |
|||
[[time perception|Chronoception]] refers to how the passage of time is perceived and experienced. Although the [[time perception|sense of time]] is not associated with a specific [[sensory system]], the work of [[psychologist]]s and [[neuroscientist]]s indicates that human brains do have a system governing the [[perception]] of [[time]],<ref name="Rao 2001">{{cite journal | vauthors = Rao SM, Mayer AR, Harrington DL | title = The evolution of brain activation during temporal processing | journal = Nature Neuroscience | volume = 4 | issue = 3 | pages = 317–23 | date = March 2001 | pmid = 11224550 | doi = 10.1038/85191 }}</ref><ref>{{cite web | url= http://www.unisci.com/stories/20011/0227013.htm | title= Brain Areas Critical To Human Time Sense Identified | access-date= | date= 2001-02-27 |publisher= UniSci – Daily University Science News}}</ref> composed of a highly distributed system involving the [[cerebral cortex]], [[cerebellum]] and [[basal ganglia]]. One particular component, the [[suprachiasmatic nucleus]], is responsible for the [[circadian rhythm|circadian (or daily) rhythm]], while other cell clusters appear to be capable of shorter-range ([[ultradian]]) timekeeping. |
|||
Two types of somatosensory signals that are transduced by [[Free nerve ending|free nerve endings]] are pain and temperature. These two modalities use [[thermoreceptor]]<nowiki/>s and [[Nociceptor|nociceptors]] to transduce temperature and pain stimuli, respectively. Temperature receptors are stimulated when local temperatures differ from [[body temperature]]. Some thermoreceptors are sensitive to just cold and others to just heat. Nociception is the sensation of potentially damaging stimuli. Mechanical, chemical, or thermal stimuli beyond a set threshold will elicit painful sensations. Stressed or damaged tissues release chemicals that activate receptor proteins in the nociceptors. For example, the sensation of heat associated with spicy foods involves [[capsaicin]], the active molecule in hot peppers.<ref name=":0" /> |
|||
One or more [[dopaminergic pathways]] in the [[central nervous system]] appear to have a strong modulatory influence on [[mental chronometry]], particularly interval timing.<ref name="Amph-DA reaction time">{{cite journal | vauthors = Parker KL, Lamichhane D, Caetano MS, Narayanan NS | title = Executive dysfunction in Parkinson's disease and timing deficits | journal = Frontiers in Integrative Neuroscience | volume = 7 | pages = 75 | date = October 2013 | pmid = 24198770 | pmc = 3813949 | doi = 10.3389/fnint.2013.00075 | quote = Manipulations of dopaminergic signaling profoundly influence interval timing, leading to the hypothesis that dopamine influences internal pacemaker, or “clock,” activity. For instance, amphetamine, which increases concentrations of dopamine at the synaptic cleft advances the start of responding during interval timing, whereas antagonists of D2 type dopamine receptors typically slow timing;... Depletion of dopamine in healthy volunteers impairs timing, while amphetamine releases synaptic dopamine and speeds up timing. }}</ref> |
|||
Low frequency vibrations are sensed by mechanoreceptors called [[Merkel cell|Merkel cells]], also known as type I cutaneous mechanoreceptors. Merkel cells are located in the [[stratum basale]] of the [[epidermis]]. Deep pressure and vibration is transduced by lamellated ([[Pacinian corpuscle|Pacinian]]) corpuscles, which are receptors with encapsulated endings found deep in the dermis, or subcutaneous tissue. Light touch is transduced by the encapsulated endings known as tactile ([[Meissner corpuscle|Meissner]]) corpuscles. Follicles are also wrapped in a [[plexus]] of nerve endings known as the hair follicle plexus. These nerve endings detect the movement of hair at the surface of the skin, such as when an insect may be walking along the [[skin]]. Stretching of the skin is transduced by stretch receptors known as [[Bulbous corpuscle|bulbous corpuscles]]. Bulbous corpuscles are also known as Ruffini corpuscles, or type II cutaneous mechanoreceptors.<ref name=":0" /> |
|||
===Agency=== |
|||
{{main | Sense of agency}} |
|||
The heat receptors are sensitive to infrared radiation and can occur in specialized organs, for instance in [[pit vipers]]. The [[Thermoreceptor|thermoceptors]] in the skin are quite different from the [[Homeostasis|homeostatic]] thermoceptors in the brain ([[POAH|hypothalamus]]), which provide feedback on internal body temperature. |
|||
The sense of agency refers to the subjective feeling of having chosen a particular action. Some conditions, such as schizophrenia, can lead to a loss of this sense, causing a person to feel like a machine or even leading to delusions of being controlled from some outside source. The opposite extreme occurs too, with some people experiencing everything in their environment as if they had decided that it would happen.<ref>{{cite book | title = The Ego Tunnel | last = Metzinger | first = Thomas | pages = 117–118 | year = 2009 | publisher = Basic Books | isbn = 978-0-465-04567-9}}</ref> |
|||
==== Vestibular system (balance) ==== |
|||
Even in non-pathological cases, there is a measurable difference between making a decision and the feeling of agency. Through methods such as [[Neuroscience of free will#Libet experiment|the Libet experiment]], a gap of half a second or more can be detected from the time when there are detectable neurological signs of a decision having been made to the time when the subject actually becomes conscious of the decision. |
|||
{{Main|Vestibular system}} |
|||
The vestibular sense, or sense of balance (equilibrium), is the sense that contributes to the perception of balance (equilibrium), spatial orientation, direction, or acceleration ([[equilibrioception]]). Along with audition, the [[inner ear]] is responsible for encoding information about equilibrium. A similar [[mechanoreceptor]]—a hair cell with [[Stereocilia (inner ear)|stereocilia]]—senses head position, head movement, and whether our bodies are in motion. These cells are located within the [[Vestibule of the ear|vestibule]] of the inner ear. Head position is sensed by the [[Utricle (ear)|utricle]] and [[saccule]], whereas head movement is sensed by the [[semicircular canals]]. The neural signals generated in the [[vestibular ganglion]] are transmitted through the [[vestibulocochlear nerve]] to the [[Brainstem|brain stem]] and [[cerebellum]].<ref name=":0" /> |
|||
There are also experiments in which an illusion of agency is induced in psychologically normal subjects. In Wegner and Wheatley 1999, subjects were given instructions to move a mouse around a scene and point to an image about once every thirty seconds. However, a second person—acting as a test subject but actually a confederate—had their hand on the mouse at the same time, and controlled some of the movement. Experimenters were able to arrange for subjects to perceive certain "forced stops" as if they were their own choice.<ref>{{cite journal | vauthors = Wegner DM, Wheatley T | title = Apparent mental causation. Sources of the experience of will | journal = The American Psychologist | volume = 54 | issue = 7 | pages = 480–92 | date = July 1999 | pmid = 10424155 | doi = 10.1037/0003-066x.54.7.480 | citeseerx = 10.1.1.188.8271 }}</ref><ref>{{cite book | last= Metzinger | first = Thomas | date= 2003 | title = Being No One | page = 508}}</ref> |
|||
The semicircular canals are three ring-like extensions of the vestibule. One is oriented in the horizontal plane, whereas the other two are oriented in the vertical plane. The [[Anatomical terms of location|anterior]] and posterior vertical canals are oriented at approximately 45 degrees relative to the [[sagittal plane]]. The base of each semicircular canal, where it meets with the vestibule, connects to an enlarged region known as the [[Ampullary cupula|ampulla]]. The ampulla contains the hair cells that respond to rotational movement, such as turning the head while saying “no.” The stereocilia of these hair cells extend into the [[Ampullary cupula|cupula]], a membrane that attaches to the top of the ampulla. As the head rotates in a plane parallel to the semicircular canal, the fluid lags, deflecting the cupula in the direction opposite to the head movement. The semicircular canals contain several ampullae, with some oriented horizontally and others oriented vertically. By comparing the relative movements of both the horizontal and vertical ampullae, the vestibular system can detect the direction of most head movements within three-dimensional ([[Three-dimensional space|3D]]) space.<ref name=":0" /> |
|||
===Familiarity=== |
|||
The [[vestibular nerve]] conducts information from sensory receptors in three [[Osseous ampullae|ampulla]] that sense motion of fluid in three [[Semicircular canal|semicircular canals]] caused by three-dimensional rotation of the head. The vestibular nerve also conducts information from the [[Utricle (ear)|utricle]] and the [[saccule]], which contain hair-like sensory receptors that bend under the weight of [[Otolith|otoliths]] (which are small crystals of [[calcium carbonate]]) that provide the inertia needed to detect head rotation, linear acceleration, and the direction of gravitational force. |
|||
[[Recognition memory]] is sometimes divided into two functions by neuroscientists: familiarity and recollection.<ref>{{cite journal | last = Mandler | year = 1980 | title = Recognizing: the judgement of prior occurrence | journal = Psychological Review | volume = 87| issue = 3 | pages = 252–271 | doi = 10.1037/0033-295X.87.3.252| url = http://www.escholarship.org/uc/item/58b2c2fc }}</ref> A strong sense of familiarity can occur without any recollection, for example in cases of [[deja vu]]. The temporal lobe, in particular the [[perirhinal cortex]], responds differently to stimuli which feel novel than to things which feel familiar. Firing rates in the perirhinal cortex are connected with the sense of familiarity in humans and other mammals. In tests, stimulating this area at 10–15 Hz caused animals to treat even novel images as familiar, and stimulation at 30–40 Hz caused novel images to be partially treated as familiar.<ref>{{cite journal | vauthors = Ho JW, Poeta DL, Jacobson TK, Zolnik TA, Neske GT, Connors BW, Burwell RD | title = Bidirectional Modulation of Recognition Memory | journal = The Journal of Neuroscience | volume = 35 | issue = 39 | pages = 13323–35 | date = September 2015 | pmid = 26424881 | pmc = 4588607 | doi = 10.1523/JNEUROSCI.2278-15.2015 }}</ref> Specifically, stimulation at 30–40 Hz led to animals looking at a familiar image for longer periods, as they would for an unfamiliar one; but it did not lead to the same exploration behavior normally associated with novelty. Recent studies on lesions in the area concluded that rats with a damaged perirhinal cortex were still more interested in exploring when novel objects were present, but seemed unable to tell novel objects from familiar ones—they examined both equally. Thus, other brain regions are involved with noticing unfamiliarity, but the perirhinal cortex is needed to associate the feeling with a specific source.<ref>{{cite journal | vauthors = Kinnavane L, Amin E, Olarte-Sánchez CM, Aggleton JP | title = Detecting and discriminating novel objects: The impact of perirhinal cortex disconnection on hippocampal activity patterns | journal = Hippocampus | volume = 26 | issue = 11 | pages = 1393–1413 | date = November 2016 | pmid = 27398938 | pmc = 5082501 | doi = 10.1002/hipo.22615 }}</ref> |
|||
==== Gustatory system (taste) ==== |
|||
{{Main|Gustatory system}} |
|||
The gustatory system or the sense of taste is the [[sensory system]] that is partially responsible for the [[perception]] of [[Flavor|taste (flavor)]].<ref>{{Cite journal|last=Trivedi|first=Bijal P.|date=2012-06|title=Gustatory system: The finer points of taste|url=http://dx.doi.org/10.1038/486s2a|journal=Nature|volume=486|issue=7403|pages=S2–S3|doi=10.1038/486s2a|issn=0028-0836}}</ref> A few recognized [[Stimulus modality|submodalities]] exist within taste: [[sweet]], [[Saltiness|salty]], [[sour]], [[Bitter (taste)|bitter]], and [[umami]]. Very recent research has suggested that there may also be a sixth taste submodality for fats, or lipids.<ref name=":0" /> The sense of taste is often confused with the perception of flavor, which is the results of the [[multimodal integration]] of gustatory (taste) and olfactory (smell) sensations.<ref>{{Cite book|last=Murray, M. M. (Micah M.), editor. Wallace, Mark T., editor.|url=http://worldcat.org/oclc/759160178|title=The neural bases of multisensory processes|isbn=978-1-4398-1219-8|oclc=759160178}}</ref> |
|||
[[File:Philippe_Mercier_-_The_Sense_of_Taste_-_Google_Art_Project.jpg|thumb|Philippe Mercier - The Sense of Taste - Google Art Project]] |
|||
Within the structure of the [[lingual papillae]] are [[Taste bud|taste buds]] that contain specialized gustatory receptor cells for the [[Transduction (physiology)|transduction]] of taste stimuli. These receptor cells are sensitive to the chemicals contained within foods that are ingested, and they release [[Neurotransmitter|neurotransmitters]] based on the amount of the chemical in the food. Neurotransmitters from the gustatory cells can activate [[Sensory neuron|sensory neurons]] in the [[Facial nerve|facial]], [[Glossopharyngeal nerve|glossopharyngeal]], and [[Vagus nerve|vagus]] [[cranial nerves]].<ref name=":0" /> |
|||
Salty and sour taste submodalities are triggered by the [[cations]] [[Na⁺|Na+]] and [[Hydrogen ion|H+]], respectively. The other taste modalities result from food molecules binding to a [[G protein-coupled receptor|G protein–coupled receptor]]. A G protein signal transduction system ultimately leads to [[depolarization]] of the gustatory cell. The sweet taste is the sensitivity of gustatory cells to the presence of [[glucose]] (or [[Sugar substitute|sugar substitutes]]) dissolved in the [[saliva]]. Bitter taste is similar to sweet in that food molecules bind to G protein–coupled receptors. The taste known as umami is often referred to as the savory taste. Like sweet and bitter, it is based on the activation of G protein–coupled receptors by a specific molecule.<ref name=":0" /> |
|||
Once the gustatory cells are activated by the taste molecules, they release [[Neurotransmitter|neurotransmitters]] onto the [[Dendrite|dendrites]] of sensory neurons. These neurons are part of the facial and glossopharyngeal cranial nerves, as well as a component within the vagus nerve dedicated to the [[Pharyngeal reflex|gag reflex]]. The facial nerve connects to taste buds in the anterior third of the tongue. The glossopharyngeal nerve connects to taste buds in the posterior two thirds of the tongue. The vagus nerve connects to taste buds in the extreme posterior of the tongue, verging on the [[pharynx]], which are more sensitive to [[Noxious stimulus|noxious stimuli]] such as bitterness.<ref name=":0" /> |
|||
Flavor depends on odor, texture, and temperature as well as on taste. Humans receive tastes through sensory organs called taste buds, or gustatory calyculi, concentrated on the upper surface of the tongue. Other tastes such as calcium<ref name="Tordoff 2008">{{cite journal|vauthors=Tordoff MG|date=August 2008|title=Gene discovery and the genetic basis of calcium consumption|journal=Physiology & Behavior|volume=94|issue=5|pages=649–59|doi=10.1016/j.physbeh.2008.04.004|pmc=2574908|pmid=18499198}}</ref><ref name="Sciencedaily 2008">{{cite web|url=https://www.sciencedaily.com/releases/2008/08/080820163008.htm|title=That Tastes ... Sweet? Sour? No, It's Definitely Calcium!|work=Sciencedaily}}</ref> and [[free fatty acids]]<ref name="Mattes 2009">{{cite journal|vauthors=Mattes RD|year=2009|title=Is there a fatty acid taste?|journal=Annual Review of Nutrition|volume=29|issue=|pages=305–27|doi=10.1146/annurev-nutr-080508-141108|pmc=2843518|pmid=19400700}}</ref> may also be basic tastes but have yet to receive widespread acceptance. The inability to taste is called [[ageusia]]. |
|||
==== Olfactory system (smell) ==== |
|||
{{Main|Olfactory system}} |
|||
Like the sense of taste, the sense of smell, or the olfactiory system, is also responsive to [[Chemical substance|chemical stimuli]].<ref name=":0" /> Unlike taste, there are hundreds of [[olfactory receptor]]<nowiki/>s (388 according to one source), each binding to a particular molecular feature. [[Odor|Odor molecules]] possess a variety of features and, thus, excite specific receptors more or less strongly. This combination of excitatory signals from different receptors makes up what humans perceive as the molecule's smell.<ref name="Moonwalk">{{cite web|url=http://sandwalk.blogspot.co.uk/2007/01/sense-of-smell-olfactory-receptors.html|title=A Sense of Smell: Olfactory Receptors|date=2007-01-09|work=Sandwalk|access-date=}}</ref> |
|||
The olfactory receptor neurons are located in a small region within the [[Nasal cavity|superior nasal cavity]]. This region is referred to as the [[olfactory epithelium]] and contains [[Bipolar neuron|bipolar sensory neurons]]. Each olfactory sensory neuron has [[Dendrite|dendrites]] that extend from the [[apical surface]] of the [[epithelium]] into the [[mucus]] lining the cavity. As airborne molecules are inhaled through the [[Human nose|nose]], they pass over the olfactory epithelial region and dissolve into the mucus. These odorant molecules bind to proteins that keep them dissolved in the mucus and help transport them to the olfactory dendrites. The odorant–protein complex binds to a receptor protein within the cell membrane of an olfactory dendrite. These receptors are G protein–coupled, and will produce a graded [[membrane potential]] in the [[Olfactory receptor neuron|olfactory neurons]].<ref name=":0" /> |
|||
[[File:The_sense_of_smell_Philippe_Mercier.jpg|thumb|The sense of smell Bequest of [[Mrs E.G. Elgar]], 1945 [[Museum of New Zealand Te Papa Tongarewa]].]] |
|||
In the [[Human brain|brain]], olfaction is processed by the [[olfactory cortex]]. Olfactory receptor neurons in the nose differ from most other neurons in that they die and regenerate on a regular basis. The inability to smell is called [[anosmia]]. Some neurons in the nose are specialized to detect [[Pheromone|pheromones]].<ref name="LiveScience">{{cite web|url=http://www.livescience.com/2737-surprising-impact-taste-smell.html|title=The Surprising Impact of Taste and Smell|work=LiveScience}}</ref> Loss of the sense of smell can result in food tasting bland. A person with an impaired sense of smell may require additional [[spice]] and [[seasoning]] levels for food to be tasted. Anosmia may also be related to some presentations of mild [[Depression (mood)|depression]], because the loss of enjoyment of food may lead to a general sense of despair. The ability of olfactory neurons to replace themselves decreases with [[Senescence|age]], leading to age-related anosmia. This explains why some [[Old age|elderly people]] salt their food more than [[Young|younger people]] do.<ref name=":0" /> |
|||
=== Internal === |
|||
{{Main|Interoception}} |
|||
==== Proprioception ==== |
|||
[[Proprioception]], the kinesthetic sense, provides the [[Parietal lobe|parietal cortex]] of the brain with information on the movement and relative positions of the parts of the body. Neurologists test this sense by telling patients to close their eyes and touch their own nose with the tip of a finger. Assuming proper proprioceptive function, at no time will the person lose awareness of where the hand actually is, even though it is not being detected by any of the other senses. Proprioception and touch are related in subtle ways, and their impairment results in surprising and deep deficits in perception and action.<ref name="roblesdelatorre">{{cite web|url=http://www.roblesdelatorre.com/gabriel/GR-IEEE-MM-2006.pdf|title=The Importance of the Sense of Touch in Virtual and Real Environments|work=International Society for Haptics|access-date=}}</ref> |
|||
==== Pain ==== |
|||
[[Nociception]] (physiological [[pain]]) signals nerve-damage or damage to tissue. The three types of pain receptors are cutaneous (skin), somatic (joints and bones), and visceral (body organs). It was previously believed that pain was simply the overloading of pressure receptors, but research in the first half of the 20th century indicated that pain is a distinct phenomenon that intertwines with all of the other senses, including touch. Pain was once considered an entirely subjective experience, but recent studies show that pain is registered in the [[anterior cingulate gyrus]] of the brain.<ref name="Fulbright 2001">{{cite journal|authors=Fulbright RK, Troche CJ, Skudlarski P, Gore JC, Wexler BE|date=November 2001|title=Functional MR imaging of regional brain activation associated with the affective experience of pain|journal=AJR. American Journal of Roentgenology|volume=177|issue=5|pages=1205–10|doi=10.2214/ajr.177.5.1771205|pmid=11641204}}</ref> The main function of pain is to attract our [[attention]] to dangers and motivate us to avoid them. For example, humans avoid touching a sharp needle, or hot object, or extending an arm beyond a safe limit because it is dangerous, and thus hurts. Without pain, people could do many dangerous things without being aware of the dangers. |
|||
==== Other internal sensations and perceptions{{anchor|Interoception}} ==== |
|||
An internal sensation and perception also known as interoception<ref name="Craig 2003">{{cite journal|vauthors=Craig AD|date=August 2003|title=Interoception: the sense of the physiological condition of the body|journal=Current Opinion in Neurobiology|volume=13|issue=4|pages=500–5|doi=10.1016/S0959-4388(03)00090-4|pmid=12965300}}</ref> is "any sense that is normally stimulated from within the body".<ref name="Dunn Galton 2010">{{cite journal|vauthors=Dunn BD, Galton HC, Morgan R, Evans D, Oliver C, Meyer M, Cusack R, Lawrence AD, Dalgleish T|date=December 2010|title=Listening to your heart. How interoception shapes emotion experience and intuitive decision making|url=https://semanticscholar.org/paper/7b2d96919d1befe4fb4ced5ba024339188d27f1c|journal=Psychological Science|volume=21|issue=12|pages=1835–44|doi=10.1177/0956797610389191|pmid=21106893}}</ref> These involve numerous sensory receptors in internal organs. Interoception is thought to be atypical in clinical conditions such as [[alexithymia]].<ref>{{cite journal|vauthors=Shah P, Hall R, Catmur C, Bird G|date=August 2016|title=Alexithymia, not autism, is associated with impaired interoception|journal=Cortex; A Journal Devoted to the Study of the Nervous System and Behavior|volume=81|pages=215–20|doi=10.1016/j.cortex.2016.03.021|pmc=4962768|pmid=27253723}}</ref> |
|||
Some examples of specific receptors are: |
|||
* [[Hunger (motivational state)|Hunger]] is governed by a set of brain structures (e.g., the [[hypothalamus]]) that are responsible for [[energy homeostasis]].<ref name="Hunger - CNS energy homeostasis">{{cite journal|vauthors=Farr OM, Li CS, Mantzoros CS|date=May 2016|title=Central nervous system regulation of eating: Insights from human brain imaging|journal=Metabolism|volume=65|issue=5|pages=699–713|doi=10.1016/j.metabol.2016.02.002|pmc=4834455|pmid=27085777}}</ref> |
|||
* [[Pulmonary stretch receptors]] are found in the lungs and control the [[respiratory rate]]. |
|||
* [[Peripheral chemoreceptors]] in the brain monitor the carbon dioxide and oxygen levels in the brain to give a perception of [[suffocation]] if carbon dioxide levels get too high.<ref name="HowStuffWorks How Your Lungs Work">{{cite web|url=http://science.howstuffworks.com/life/human-biology/lung3.htm|title=How Your Lungs Work|date=2000-10-06|work=HowStuffWorks}}</ref> |
|||
* The [[chemoreceptor trigger zone]] is an area of the [[Medulla oblongata|medulla]] in the brain that receives inputs from [[blood]]-borne [[Drug|drugs]] or [[Hormone|hormones]], and communicates with the [[vomiting center]]. |
|||
* Chemoreceptors in the circulatory system also measure salt levels and prompt thirst if they get too high; they can also respond to high [[blood sugar]] levels in diabetics. |
|||
* [[Cutaneous receptor|Cutaneous receptors]] in the skin not only respond to touch, pressure, temperature and vibration, but also respond to vasodilation in the skin such as [[blushing]]. |
|||
* Stretch receptors in the [[gastrointestinal tract]] sense gas distension that may result in colic pain. |
|||
* Stimulation of sensory receptors in the [[esophagus]] result in sensations felt in the throat when [[swallowing]], [[vomiting]], or during [[acid reflux]]. |
|||
* Sensory receptors in [[pharynx]] mucosa, similar to touch receptors in the skin, sense foreign objects such as mucous and food that may result in a [[gag reflex]] and corresponding gagging sensation. |
|||
* Stimulation of sensory receptors in the [[urinary bladder]] and [[rectum]] may result in perceptions of fullness. |
|||
* Stimulation of stretch sensors that sense dilation of various blood vessels may result in pain, for example headache caused by vasodilation of brain arteries. |
|||
* Cardioception refers to the perception of the activity of the heart.<ref>{{cite journal|vauthors=Garfinkel SN, Seth AK, Barrett AB, Suzuki K, Critchley HD|date=January 2015|title=Knowing your own heart: distinguishing interoceptive accuracy from interoceptive awareness|journal=Biological Psychology|volume=104|pages=65–74|doi=10.1016/j.biopsycho.2014.11.004|pmid=25451381}}</ref><ref>{{cite journal|vauthors=Schandry R|date=July 1981|title=Heart beat perception and emotional experience|journal=Psychophysiology|volume=18|issue=4|pages=483–8|doi=10.1111/j.1469-8986.1981.tb02486.x|pmid=7267933}}</ref><ref>{{cite journal|vauthors=Kleckner IR, Wormwood JB, Simmons WK, Barrett LF, Quigley KS|date=November 2015|title=Methodological recommendations for a heartbeat detection-based measure of interoceptive sensitivity|journal=Psychophysiology|volume=52|issue=11|pages=1432–40|doi=10.1111/psyp.12503|pmc=4821012|pmid=26265009}}</ref><ref>{{cite journal|vauthors=Whitehead WE, Drescher VM, Heiman P, Blackwell B|date=December 1977|title=Relation of heart rate control to heartbeat perception|journal=Biofeedback and Self-Regulation|volume=2|issue=4|pages=317–92|doi=10.1007/BF00998623|pmid=612350}}</ref> |
|||
* [[Opsin|Opsins]] and [[direct DNA damage]] in [[Melanocyte|melanocytes]] and [[Keratinocyte|keratinocytes]] can sense [[ultraviolet]] radiation, which plays a role in pigmentation and [[sunburn]]. |
|||
* [[Baroreceptor|Baroreceptors]] relay blood pressure information to the brain and maintain proper homeostatic blood pressure. |
|||
== Nonhuman animal sensation and perception == |
|||
==Non-human== |
|||
=== |
=== Human analogues === |
||
Other living organisms have receptors to sense the world around them, including many of the senses listed above for humans. However, the mechanisms and capabilities vary widely. |
Other living organisms have receptors to sense the world around them, including many of the senses listed above for humans. However, the mechanisms and capabilities vary widely. |
||
====Smell==== |
==== Smell ==== |
||
An example of smell in non-mammals is that of [[sharks]], which combine their keen sense of smell with timing to determine the direction of a smell. They follow the nostril that first detected the smell.<ref name="Gardiner Atema 2010">{{cite journal |
An example of smell in non-mammals is that of [[sharks]], which combine their keen sense of smell with timing to determine the direction of a smell. They follow the nostril that first detected the smell.<ref name="Gardiner Atema 2010">{{cite journal|vauthors=Gardiner JM, Atema J|date=July 2010|title=The function of bilateral odor arrival time differences in olfactory orientation of sharks|journal=Current Biology|volume=20|issue=13|pages=1187–91|bibcode=1996CBio....6.1213A|doi=10.1016/j.cub.2010.04.053|pmid=20541411}}</ref> [[Insect|Insects]] have olfactory receptors on their [[Antenna (biology)|antennae]]. Although it is unknown to the degree and magnitude which non-human animals can smell better than humans.<ref>{{Cite news|last=Devlin|first=Hannah|url=https://www.theguardian.com/science/2017/may/11/not-to-be-sniffed-at-human-sense-of-smell-rivals-that-of-dogs-says-study|title=Not to be sniffed at: human sense of smell rivals that of dogs, says study|date=2017-05-11|work=The Guardian|access-date=2019-04-10|issn=0261-3077|name-list-format=vanc}}</ref> |
||
====Vomeronasal organ==== |
==== Vomeronasal organ ==== |
||
Many animals ([[ |
Many animals ([[Salamander|salamanders]], [[Reptile|reptiles]], [[Mammal|mammals]]) have a [[vomeronasal organ]]<ref name="Takami 2002">{{cite journal|vauthors=Takami S|date=August 2002|title=Recent progress in the neurobiology of the vomeronasal organ|journal=Microscopy Research and Technique|volume=58|issue=3|pages=228–50|doi=10.1002/jemt.10094|pmid=12203701}}</ref> that is connected with the mouth cavity. In mammals it is mainly used to detect [[pheromones]] of marked territory, trails, and sexual state. Reptiles like [[Snake|snakes]] and [[Monitor lizard|monitor lizards]] make extensive use of it as a smelling organ by transferring scent molecules to the vomeronasal organ with the tips of the forked tongue. In reptiles the vomeronasal organ is commonly referred to as Jacobsons organ. In mammals, it is often associated with a special behavior called [[flehmen]] characterized by uplifting of the lips. The organ is [[Human vestigiality|vestigial in humans]], because associated neurons have not been found that give any sensory input in humans.<ref name="Frasnelli 2011">{{cite journal|vauthors=Frasnelli J, Lundström JN, Boyle JA, Katsarkas A, Jones-Gotman M|date=March 2011|title=The vomeronasal organ is not involved in the perception of endogenous odors|journal=Human Brain Mapping|volume=32|issue=3|pages=450–60|doi=10.1002/hbm.21035|pmc=3607301|pmid=20578170}}</ref> |
||
====Taste==== |
==== Taste ==== |
||
[[Flies]] and [[butterflies]] have taste organs on their feet, allowing them to taste anything they land on. [[Catfish]] have taste organs across their entire bodies, and can taste anything they touch, including chemicals in the water.<ref>Atema, Jelle (1980) [https://books.google.com/books?id=TZbRp0z5-p0C&pg=PA57 "Chemical senses, chemical signals, and feeding behavior in fishes"] p. 57–101. In: Bardach, JE ''Fish behavior and its use in the capture and culture of fishes', The WorldFish Center, {{ISBN|978-971-02-0003-0}}.</ref> |
[[Flies]] and [[butterflies]] have taste organs on their feet, allowing them to taste anything they land on. [[Catfish]] have taste organs across their entire bodies, and can taste anything they touch, including chemicals in the water.<ref>Atema, Jelle (1980) [https://books.google.com/books?id=TZbRp0z5-p0C&pg=PA57 "Chemical senses, chemical signals, and feeding behavior in fishes"] p. 57–101. In: Bardach, JE ''Fish behavior and its use in the capture and culture of fishes', The WorldFish Center, {{ISBN|978-971-02-0003-0}}.''</ref> |
||
====Vision |
==== Vision ==== |
||
[[Cat]] |
[[Cat|Cats]] have the ability to see in low light, which is due to muscles surrounding their [[Iris (anatomy)|irides]]–which contract and expand their pupils–as well as to the [[tapetum lucidum]], a reflective membrane that optimizes the image. |
||
[[Crotalinae|Pit vipers]], [[Pythonidae|pythons]] and some [[Boidae| |
[[Crotalinae|Pit vipers]], [[Pythonidae|pythons]] and some [[Boidae|boas]] have organs that allow them to detect [[infrared]] light, such that these snakes are able to sense the body heat of their prey. The [[common vampire bat]] may also have an infrared sensor on its nose.<ref>{{cite web|url=http://www.pitt.edu/AFShome/s/l/slavic/public/html/courses/vampires/images/bats/vambat.html|title=The illustrated story of the Vampire bat|access-date=2007-05-25}}</ref> It has been found that [[birds]] and some other animals are [[tetrachromats]] and have the ability to see in the [[ultraviolet]] down to 300 nanometers. [[Bees]] and [[dragonflies]]<ref name="van Kleef Berry 2008">{{cite journal|vauthors=van Kleef J, Berry R, Stange G|date=March 2008|title=Directional selectivity in the simple eye of an insect|journal=The Journal of Neuroscience|volume=28|issue=11|pages=2845–55|doi=10.1523/JNEUROSCI.5556-07.2008|pmc=6670670|pmid=18337415}}</ref> are also able to see in the ultraviolet. [[Mantis shrimp|Mantis shrimps]] can perceive both [[polarized light]] and [[multispectral]] images and have twelve distinct kinds of color receptors, unlike humans which have three kinds and most mammals which have two kinds.<ref>{{cite journal|vauthors=Marshall J, Oberwinkler J|date=October 1999|title=The colourful world of the mantis shrimp|journal=Nature|volume=401|issue=6756|pages=873–4|bibcode=1999Natur.401..873M|doi=10.1038/44751|pmid=10553902}}</ref> |
||
[[Cephalopod]] |
[[Cephalopod|Cephalopods]] have the ability to change color using [[Chromatophore|chromatophores]] in their skin. Researchers believe that [[Opsin|opsins]] in the skin can sense different wavelengths of light and help the creatures choose a coloration that camouflages them, in addition to light input from the eyes.<ref>[http://thedishonscience.stanford.edu/posts/octopus-vision-is-in-the-skin-of-the-beholder/ Octopus vision, it's in the eye (or skin) of the beholder]</ref> Other researchers hypothesize that [[Cephalopod eye|cephalopod eyes]] in species which only have a single [[photoreceptor protein]] may use [[chromatic aberration]] to turn monochromatic vision into color vision,<ref>[https://phys.org/news/2016-07-explanation-cephalopods-black-white-vision.html Study proposes explanation for how cephalopods see color, despite black and white vision]</ref> explaining pupils shaped like the letter U, the letter W, or a [[dumbbell]], as well as explaining the need for colorful mating displays.<ref>[https://www.futurity.org/octopus-color-pupils-1196262/ Odd pupils let ‘colorblind’ octopuses see colors]</ref> Some cephalopods can distinguish the polarization of light. |
||
==== |
==== Spatial orientation ==== |
||
Many invertebrates have a [[statocyst]], which is a sensor for acceleration and orientation that works very differently from the mammalian's semi-circular canals. |
Many invertebrates have a [[statocyst]], which is a sensor for acceleration and orientation that works very differently from the mammalian's semi-circular canals. |
||
===Not |
=== Not human analogues === |
||
In addition, some animals have senses that humans do not, including the following: |
In addition, some animals have senses that humans do not, including the following: |
||
==== Magnetoception ==== |
|||
[[Magnetoception]] (or magnetoreception) is the ability to detect the direction one is facing based on the Earth's [[magnetic field]]. Directional awareness is most commonly observed in [[Bird|birds]], which rely on their magnetic sense to navigate during migration.<ref name="Theoretical and Computational Biophysics Group">{{cite web|url=http://www.ks.uiuc.edu/Research/magsense/ms.html|title=The Magnetic Sense of Animals|work=Theoretical and Computational Biophysics Group}}</ref><ref name="Theoretical and Computational Biophysics Group" /><ref name="Baylor College of Medicine">{{cite web|url=http://www.bcm.edu/news/item.cfm?newsID=5575|title=Built-in GPS in birds in tune with Earth's magnetic field|work=Baylor College of Medicine|access-date=}}</ref>{{Dead link|date=May 2019|fix-attempted=yes}}<ref name="Wu Dickman 2012">{{cite journal|vauthors=Wu LQ, Dickman JD|date=May 2012|title=Neural correlates of a magnetic sense|journal=Science|volume=336|issue=6084|pages=1054–7|bibcode=2012Sci...336.1054W|doi=10.1126/science.1216567|pmid=22539554}}</ref><ref name="Cressey2012">{{cite journal|last1=Cressey|first1=Daniel|year=2012|title=Pigeons may 'hear' magnetic fields|journal=Nature|doi=10.1038/nature.2012.10540|issn=1744-7933|name-list-format=vanc}}</ref> It has also been observed in insects such as [[Bee|bees]]. Cattle make use of magnetoception to align themselves in a north-south direction.<ref name="BBC News - Science/Nature">{{cite news|url=http://news.bbc.co.uk/1/hi/sci/tech/7575459.stm|title=Cattle shown to align north-south|work=BBC News - Science/Nature}}</ref> [[Magnetotactic bacteria]] build miniature magnets inside themselves and use them to determine their orientation relative to the Earth's magnetic field.<ref name="Blakemore 1975">{{cite journal|vauthors=Blakemore R|date=October 1975|title=Magnetotactic bacteria|journal=Science|volume=190|issue=4212|pages=377–9|bibcode=1975Sci...190..377B|doi=10.1126/science.170679|pmid=170679}}</ref><ref name="Urban 2000">{{cite journal|vauthors=Urban JE|date=November 2000|title=Adverse effects of microgravity on the magnetotactic bacterium Magnetospirillum magnetotacticum|journal=Acta Astronautica|volume=47|issue=10|pages=775–80|bibcode=2000AcAau..47..775U|doi=10.1016/S0094-5765(00)00120-X|pmid=11543576}}</ref> There has been some recent (tentative) research suggesting that the [[Rhodopsin]] in the human eye, which responds particularly well to blue light, can facilitate magnetoception in humans.<ref>{{cite journal|vauthors=Chae KS, Oh IT, Lee SH, Kim SC|date=2019-02-14|title=Blue light-dependent human magnetoreception in geomagnetic food orientation|journal=PLOS ONE|volume=14|issue=2|pages=e0211826|doi=10.1371/journal.pone.0211826|pmc=6375564|pmid=30763322}}</ref> |
|||
==== Echolocation ==== |
==== Echolocation ==== |
||
{{Main|Animal echolocation}} |
{{Main|Animal echolocation}} |
||
Certain animals, including [[ |
Certain animals, including [[Bat|bats]] and [[Cetacea|cetaceans]], have the ability to determine orientation to other objects through interpretation of reflected sound (like [[sonar]]). They most often use this to navigate through poor lighting conditions or to identify and track prey. There is currently an uncertainty whether this is simply an extremely developed post-sensory interpretation of auditory perceptions or it actually constitutes a separate sense. Resolution of the issue will require brain scans of animals while they actually perform echolocation, a task that has proven difficult in practice. |
||
Blind people report they are able to navigate and in some cases identify an object by interpreting reflected sounds (especially their own footsteps), a phenomenon known as [[human echolocation]]. |
Blind people report they are able to navigate and in some cases identify an object by interpreting reflected sounds (especially their own footsteps), a phenomenon known as [[human echolocation]]. |
||
==== Electroreception ==== |
==== Electroreception ==== |
||
[[Electroreception]] (or electroception) is the ability to detect [[ |
[[Electroreception]] (or electroception) is the ability to detect [[Electric field|electric fields]]. Several species of fish, [[Shark|sharks]], and rays have the capacity to sense changes in electric fields in their immediate vicinity. For cartilaginous fish this occurs through a specialized organ called the [[Ampullae of Lorenzini]]. Some fish passively sense changing nearby electric fields; some generate their own weak electric fields, and sense the pattern of field potentials over their body surface; and some use these electric field generating and sensing capacities for social [[communication]]. The mechanisms by which electroceptive fish construct a spatial representation from very small differences in field potentials involve comparisons of spike latencies from different parts of the fish's body. |
||
The only orders of mammals that are known to demonstrate electroception are the [[dolphin]] and [[monotreme]] orders. Among these mammals, the [[platypus]]<ref name="Electroreceptive Mechanisms in the Platypus">{{cite web |
The only orders of mammals that are known to demonstrate electroception are the [[dolphin]] and [[monotreme]] orders. Among these mammals, the [[platypus]]<ref name="Electroreceptive Mechanisms in the Platypus">{{cite web|url=http://instruct1.cit.cornell.edu/courses/bionb420.07/anelson/electrorecept.html|title=Electroreceptive Mechanisms in the Platypus|website=|archive-url=https://web.archive.org/web/19990209085517/http://instruct1.cit.cornell.edu/courses/bionb420.07/anelson/electrorecept.html|archive-date=1999-02-09|access-date=}}</ref> has the most acute sense of electroception. |
||
A dolphin can detect electric fields in water using electroreceptors in [[Whisker|vibrissal crypts]] arrayed in pairs on its snout and which evolved from whisker motion sensors.<ref name="Drake 2011">{{cite journal|last1=Drake|first1=Nadia |
A dolphin can detect electric fields in water using electroreceptors in [[Whisker|vibrissal crypts]] arrayed in pairs on its snout and which evolved from whisker motion sensors.<ref name="Drake 2011">{{cite journal|last1=Drake|first1=Nadia|year=2011|title=Life: Dolphin can sense electric fields: Ability may help species track prey in murky waters|journal=Science News|volume=180|issue=5|pages=12|doi=10.1002/scin.5591800512|name-list-format=vanc}}</ref> These electroreceptors can detect electric fields as weak as 4.6 microvolts per centimeter, such as those generated by contracting muscles and pumping gills of potential prey. This permits the dolphin to locate prey from the seafloor where sediment limits visibility and echolocation. |
||
Spiders have been shown to detect electric fields to determine a suitable time to extend web for 'ballooning'.<ref>{{cite journal |
Spiders have been shown to detect electric fields to determine a suitable time to extend web for 'ballooning'.<ref>{{cite journal|last1=Morley|first1=Erica|date=July 5, 2018|title=Electric Fields Elicit Ballooning in Spiders|journal=Current Biology|volume=28|issue=14}}</ref> |
||
[[Body modification]] enthusiasts have experimented with magnetic implants to attempt to replicate this sense.<ref>{{cite web|url=http://www.boingboing.net/2005/05/05/implant-gives-man-th.html|title=Implant gives man the sense of "magnetic vision" |
[[Body modification]] enthusiasts have experimented with magnetic implants to attempt to replicate this sense.<ref>{{cite web|url=http://www.boingboing.net/2005/05/05/implant-gives-man-th.html|title=Implant gives man the sense of "magnetic vision"|access-date=2011-04-23}}</ref> However, in general humans (and it is presumed other mammals) can detect electric fields only indirectly by detecting the effect they have on hairs. An electrically charged balloon, for instance, will exert a force on human arm hairs, which can be felt through tactition and identified as coming from a static charge (and not from wind or the like). This is not electroreception, as it is a post-sensory cognitive action. |
||
====Hygroreception==== |
==== Hygroreception ==== |
||
[[Hygroreception]] is the ability to detect changes in the moisture content of the environment.<ref>{{cite journal |
[[Hygroreception]] is the ability to detect changes in the moisture content of the environment.<ref name=":2">{{cite journal|vauthors=Enjin A, Zaharieva EE, Frank DD, Mansourian S, Suh GS, Gallio M, Stensmyr MC|date=May 2016|title=Humidity Sensing in Drosophila|journal=Current Biology|volume=26|issue=10|pages=1352–8|bibcode=1996CBio....6.1213A|doi=10.1016/j.cub.2016.03.049|pmc=5305172|pmid=27161501}}</ref><ref>{{cite journal|vauthors=Tichy H, Kallina W|date=2013-01-16|title=The evaporative function of cockroach hygroreceptors|journal=PLOS ONE|volume=8|issue=1|pages=e53998|bibcode=2013PLoSO...853998T|doi=10.1371/journal.pone.0053998|pmc=3546976|pmid=23342058}}</ref> |
||
====Infrared sensing==== |
==== Infrared sensing ==== |
||
{{Main|Infrared sensing in snakes}} |
{{Main|Infrared sensing in snakes}} |
||
The ability to sense [[infrared]] thermal radiation evolved independently in various families of [[snakes]]. Essentially, it allows these reptiles to "see" radiant heat at [[wavelengths]] between 5 and 30 [[μm]] to a degree of accuracy such that a blind [[rattlesnake]] can target vulnerable body parts of the prey at which it strikes.<ref>(Kardong & Mackessy 1991)</ref> It was previously thought that the organs evolved primarily as prey detectors, but it is now believed that it may also be used in thermoregulatory decision making.<ref>(Krochmal et al. 2004)</ref> The facial pit underwent [[parallel evolution]] in [[pitvipers]] and some [[Boidae|boas]] and [[pythons]], having evolved once in pitvipers and multiple times in boas and pythons.<ref>(Pough et al. 1992)</ref> The [[electrophysiology]] of the structure is similar between the two lineages, but they differ in gross structural [[anatomy]]. Most superficially, pitvipers possess one large pit organ on either side of the head, between the eye and the nostril ([[Loreal pit]]), while boas and pythons have three or more comparatively smaller pits lining the upper and sometimes the lower lip, in or between the scales. Those of the pitvipers are the more advanced, having a suspended sensory membrane as opposed to a simple pit structure. Within the family [[Viperidae]], the pit organ is seen only in the subfamily Crotalinae: the pitvipers. The organ is used extensively to detect and target [[endothermic]] prey such as rodents and birds, and it was previously assumed that the organ evolved specifically for that purpose. However, recent evidence shows that the pit organ may also be used for thermoregulation. According to Krochmal et al., pitvipers can use their pits for thermoregulatory decision-making while true vipers (vipers who do not contain heat-sensing pits) cannot. |
The ability to sense [[infrared]] thermal radiation evolved independently in various families of [[snakes]]. Essentially, it allows these reptiles to "see" radiant heat at [[wavelengths]] between 5 and 30 [[μm]] to a degree of accuracy such that a blind [[rattlesnake]] can target vulnerable body parts of the prey at which it strikes.<ref>(Kardong & Mackessy 1991)</ref> It was previously thought that the organs evolved primarily as prey detectors, but it is now believed that it may also be used in thermoregulatory decision making.<ref>(Krochmal et al. 2004)</ref> The facial pit underwent [[parallel evolution]] in [[pitvipers]] and some [[Boidae|boas]] and [[pythons]], having evolved once in pitvipers and multiple times in boas and pythons.<ref>(Pough et al. 1992)</ref> The [[electrophysiology]] of the structure is similar between the two lineages, but they differ in gross structural [[anatomy]]. Most superficially, pitvipers possess one large pit organ on either side of the head, between the eye and the nostril ([[Loreal pit]]), while boas and pythons have three or more comparatively smaller pits lining the upper and sometimes the lower lip, in or between the scales. Those of the pitvipers are the more advanced, having a suspended sensory membrane as opposed to a simple pit structure. Within the family [[Viperidae]], the pit organ is seen only in the subfamily Crotalinae: the pitvipers. The organ is used extensively to detect and target [[endothermic]] prey such as rodents and birds, and it was previously assumed that the organ evolved specifically for that purpose. However, recent evidence shows that the pit organ may also be used for thermoregulation. According to Krochmal et al., pitvipers can use their pits for thermoregulatory decision-making while true vipers (vipers who do not contain heat-sensing pits) cannot. |
||
In spite of its detection of IR light, the pits' IR detection mechanism is not similar to photoreceptors – while photoreceptors detect light via photochemical reactions, the protein in the pits of snakes is in fact a temperature-sensitive ion channel. It senses infrared signals through a mechanism involving warming of the pit organ, rather than a chemical reaction to light.<ref name="Gracheva et al. 2010">(Gracheva et al. 2010)</ref> This is consistent with the thin pit membrane, which allows incoming IR radiation to quickly and precisely warm a given ion channel and trigger a nerve impulse, as well as vascularize the pit membrane in order to rapidly cool the ion channel back to its original "resting" or "inactive" temperature.<ref name="Gracheva et al. 2010"/> |
In spite of its detection of IR light, the pits' IR detection mechanism is not similar to photoreceptors – while photoreceptors detect light via photochemical reactions, the protein in the pits of snakes is in fact a temperature-sensitive ion channel. It senses infrared signals through a mechanism involving warming of the pit organ, rather than a chemical reaction to light.<ref name="Gracheva et al. 2010">(Gracheva et al. 2010)</ref> This is consistent with the thin pit membrane, which allows incoming IR radiation to quickly and precisely warm a given ion channel and trigger a nerve impulse, as well as vascularize the pit membrane in order to rapidly cool the ion channel back to its original "resting" or "inactive" temperature.<ref name="Gracheva et al. 2010" /> |
||
==== Other ==== |
==== Other ==== |
||
Pressure detection uses the organ of Weber, a system consisting of three appendages of vertebrae transferring changes in shape of the [[gas bladder]] to the middle ear. It can be used to regulate the buoyancy of the fish. Fish like the [[weather fish]] and other loaches are also known to respond to low pressure areas but they lack a swim bladder. |
|||
* Current detection is a detection system of water currents, consisting mostly of [[vortex|vortices]], found in the [[lateral line]] of fish and aquatic forms of amphibians. The lateral line is also sensitive to low-frequency vibrations. The mechanoreceptors are [[hair cell]]s, the same mechanoreceptors for vestibular sense and hearing. It is used primarily for navigation, hunting, and schooling. The receptors of the [[electroception|electrical sense]] are modified hair cells of the lateral line system. |
|||
*[[Polarized light]] direction/detection is used by [[bees]] to orient themselves, especially on cloudy days. [[Cuttlefish]], some [[beetles]], and [[mantis shrimp]] can also perceive the polarization of light. Most sighted humans can in fact learn to roughly detect large areas of polarization by an effect called [[Haidinger's brush]], however this is considered an [[entoptic phenomenon]] rather than a separate sense. |
|||
* [[Slit sensilla]]e of spiders detect mechanical strain in the exoskeleton, providing information on force and vibrations. |
|||
Current detection is a detection system of water currents, consisting mostly of [[Vortex|vortices]], found in the [[lateral line]] of fish and aquatic forms of amphibians. The lateral line is also sensitive to low-frequency vibrations. The mechanoreceptors are [[Hair cell|hair cells]], the same mechanoreceptors for vestibular sense and hearing. It is used primarily for navigation, hunting, and schooling. The receptors of the [[Electroception|electrical sense]] are modified hair cells of the lateral line system. |
|||
===Plant senses=== |
|||
[[Polarized light]] direction/detection is used by [[bees]] to orient themselves, especially on cloudy days. [[Cuttlefish]], some [[beetles]], and [[mantis shrimp]] can also perceive the polarization of light. Most sighted humans can in fact learn to roughly detect large areas of polarization by an effect called [[Haidinger's brush]], however this is considered an [[entoptic phenomenon]] rather than a separate sense. |
|||
[[Slit sensilla|Slit sensillae]] of spiders detect mechanical strain in the exoskeleton, providing information on force and vibrations. |
|||
== Plant sensation == |
|||
By using a variety of sense receptors, plants sense light, temperature, humidity, chemical substances, chemical gradients, reorientation, magnetic fields, infections, tissue damage and mechanical pressure. The absence of a nervous system notwithstanding, plants interpret and respond to these stimuli by a variety of hormonal and cell-to-cell communication pathways that result in movement, morphological changes and physiological state alterations at the organism level, that is, result in plant behavior. Such physiological and cognitive functions are generally not believed to give rise to mental phenomena or qualia, however, as these are typically considered the product of nervous system activity. The emergence of mental phenomena from the activity of systems functionally or computationally analogous to that of nervous systems is, however, a hypothetical possibility explored by some schools of thought in the philosophy of mind field, such as [[Functionalism (philosophy of mind)|functionalism]] and [[Computational theory of mind|computationalism]]. |
By using a variety of sense receptors, plants sense light, temperature, humidity, chemical substances, chemical gradients, reorientation, magnetic fields, infections, tissue damage and mechanical pressure. The absence of a nervous system notwithstanding, plants interpret and respond to these stimuli by a variety of hormonal and cell-to-cell communication pathways that result in movement, morphological changes and physiological state alterations at the organism level, that is, result in plant behavior. Such physiological and cognitive functions are generally not believed to give rise to mental phenomena or qualia, however, as these are typically considered the product of nervous system activity. The emergence of mental phenomena from the activity of systems functionally or computationally analogous to that of nervous systems is, however, a hypothetical possibility explored by some schools of thought in the philosophy of mind field, such as [[Functionalism (philosophy of mind)|functionalism]] and [[Computational theory of mind|computationalism]]. |
||
However, plants could perceive the world around them,<ref>{{cite web|url=http://www.bbc.com/earth/story/20170109-plants-can-see-hear-and-smell-and-respond|title=Earth - Plants can see, hear and smell – and respond |
However, plants could perceive the world around them,<ref name=":3">{{cite web|url=http://www.bbc.com/earth/story/20170109-plants-can-see-hear-and-smell-and-respond|title=Earth - Plants can see, hear and smell – and respond|date=10 January 2017|website=BBC}}</ref> and might be able to emit airborne sounds similar to "screaming" when [[Stress (biology)|stressed]]. Those noises could not be detectable by human ears, but organisms with a [[hearing range]] that can hear [[Ultrasonic|ultrasonic frequencies]]—like mice, bats or perhaps other plants—could hear the plants' cries from as far as {{convert|15|ft}} away.<ref>{{cite journal|author1=I. Khait|author2=O. Lewin-Epstein|author3=R. Sharon|author4=K. Saban|author5=R. Perelman|author6=A. Boonman|author7=Y. Yovel|author8=L. Hadany|year=2 December 2019|title=Plants emit informative airborne sounds under stress|url=https://www.biorxiv.org/content/biorxiv/early/2019/12/02/507590.full.pdf|publisher=bioRxiv|doi=10.1101/507590}}</ref> |
||
== Artificial sensation and perception == |
|||
==Culture== |
|||
{{Main|Machine perception}} |
|||
{{Further|Five wits|Ṣaḍāyatana|Ayatana|Indriya}} |
|||
Machine perception is the capability of a [[Computer System|computer system]] to interpret [[data]] in a manner that is similar to the way humans use their senses to relate to the world around them.<ref name=":4" /><ref name=":5" /><ref>{{Cite web|url=http://www.ccs.fau.edu/~hahn/mpcr/|title=Machine Perception & Cognitive Robotics Laboratory|website=www.ccs.fau.edu|access-date=2016-06-18}}</ref> Computers take in and respond to their environment through attached [[Computer hardware|hardware]]. Until recently, input was limited to a keyboard, or a mouse, but advances in technology, both in hardware and software, have allowed computers to take in [[Stimulus (physiology)|sensory input]] in a way similar to humans.<ref name=":4" /><ref name=":5" /> |
|||
[[File:1668 Gérard de Lairesse - Allegory of the Five Senses.jpg|thumb|[[Gérard de Lairesse|Lairesse's]] ''Allegory of the Five Senses'']] |
|||
== Culture == |
|||
In the time of [[William Shakespeare]], there were commonly reckoned to be five wits or five senses.<ref name=Furness1>{{cite book|chapter=King Lear|title=Shakespeare|page=187|location=Philadelphia|publisher=J.B. Lippincott Co.|oclc=1932507|author=Horace Howard Furness|volume=5|edition=7th|year=1880|author-link=Horace Howard Furness}}</ref> At that time, the words "sense" and "wit" were synonyms,<ref name=Furness1 /> so the senses were known as the five outward wits.<ref>{{cite encyclopaedia|article=wit|encyclopedia=The Merriam-Webster new book of word histories|publisher=Merriam-Webster|date=1991|isbn=978-0-87779-603-9|oclc=24246335|pages=[https://archive.org/details/merriamwebsterne00merr/page/508 508]|url-access=registration|url=https://archive.org/details/merriamwebsterne00merr/page/508}}</ref><ref>{{cite book|title=Studies in Words|author=Clive Staples Lewis|edition=2nd (republished)|publisher=Cambridge University Press|year=1990|isbn=978-0-521-39831-2|oclc=489987083|page=147|chapter=Sense|author-link=C. S. Lewis}}</ref> This traditional concept of five senses is common today. |
|||
{{Further|Five wits|Āyatana|Indriya|}} |
|||
{{Cleanup section|reason=This section may contain original research. Additional citations are needed.|date=2020-03-03}} |
|||
[[File:1668_Gérard_de_Lairesse_-_Allegory_of_the_Five_Senses.jpg|thumb|[[Gérard de Lairesse|Lairesse's]]]] |
|||
[[File:Peacock_served_in_full_plumage_(detail_of_BRUEGHEL_Taste,_Hearing_and_Touch).jpg|thumb|Detail of ''The Senses of Hearing, Touch and Taste'', [[Jan Brueghel the Elder]], 1618]] |
|||
[[File:Pietro_Paolini_-_Allegory_of_the_Five_Senses_-_Walters_372768.jpg|thumb|In this painting by [[Pietro Paolini]], each individual represents one of the five senses.<ref>{{cite web|url=http://art.thewalters.org/detail/15244|title=Allegory of the Five Senses|publisher=[[The Walters Art Museum]]}}</ref>]] |
|||
In the time of [[William Shakespeare]], there were commonly reckoned to be five wits or five senses.<ref name="Furness1">{{cite book|author=Horace Howard Furness|title=Shakespeare|publisher=J.B. Lippincott Co.|year=1880|edition=7th|volume=5|location=Philadelphia|page=187|chapter=King Lear|oclc=1932507|author-link=Horace Howard Furness}}</ref> At that time, the words "sense" and "wit" were synonyms,<ref name="Furness1" /> so the senses were known as the five outward wits.<ref>{{cite encyclopaedia|article=wit|encyclopedia=The Merriam-Webster new book of word histories|publisher=Merriam-Webster|date=1991|isbn=978-0-87779-603-9|oclc=24246335|pages=[https://archive.org/details/merriamwebsterne00merr/page/508 508]|url-access=registration|url=https://archive.org/details/merriamwebsterne00merr/page/508}}</ref><ref>{{cite book|author=Clive Staples Lewis|title=Studies in Words|publisher=Cambridge University Press|year=1990|isbn=978-0-521-39831-2|edition=2nd (republished)|page=147|chapter=Sense|oclc=489987083|author-link=C. S. Lewis}}</ref> This traditional concept of five senses is common today. |
|||
The traditional five senses are enumerated as the "five material faculties" (''{{IAST|pañcannaṃ indriyānaṃ avakanti}}'') in Hindu literature. They appear in allegorical representation as early as in the [[Katha Upanishad]] (roughly 6th century BC), as five horses drawing the "[[chariot]]" of the body, guided by the mind as "chariot driver". |
The traditional five senses are enumerated as the "five material faculties" (''{{IAST|pañcannaṃ indriyānaṃ avakanti}}'') in Hindu literature. They appear in allegorical representation as early as in the [[Katha Upanishad]] (roughly 6th century BC), as five horses drawing the "[[chariot]]" of the body, guided by the mind as "chariot driver". |
||
Depictions of the five traditional senses as [[allegory]] became a popular subject for seventeenth-century artists, especially among [[Dutch Golden Age painting|Dutch]] and [[Flemish Baroque painter]] |
Depictions of the five traditional senses as [[allegory]] became a popular subject for seventeenth-century artists, especially among [[Dutch Golden Age painting|Dutch]] and [[Flemish Baroque painter|Flemish Baroque painters]]. A typical example is [[Gérard de Lairesse]]'s ''Allegory of the Five Senses'' (1668), in which each of the figures in the main group alludes to a sense: Sight is the reclining boy with a [[convex mirror]], hearing is the [[cupid]]-like boy with a [[Triangle (musical instrument)|triangle]], smell is represented by the girl with flowers, taste is represented by the woman with the fruit, and touch is represented by the woman holding the bird. |
||
In [[Buddhist philosophy]], [[Ayatana]] or "sense-base" includes the mind as a sense organ, in addition to the traditional five. This addition to the commonly acknowledged senses may arise from the psychological orientation involved in Buddhist thought and practice. The mind considered by itself is seen as the principal gateway to a different spectrum of phenomena that differ from the physical sense data. This way of viewing the human sense system indicates the importance of internal sources of sensation and perception that complements our experience of the external world.{{Citation needed|date=March 2020}} |
|||
== See also == |
== See also == |
||
Line 244: | Line 400: | ||
* The 2004 [[Nobel Prize]] in [[Nobel Prize in Physiology or Medicine|Physiology or Medicine]] ([http://nobelprize.org/medicine/laureates/2004/index.html announced] 4 October 2004) was won by [[Richard Axel]] and [[Linda Buck]] for their work explaining olfaction, published first in a joint paper in 1991 that described the very large family of about one thousand genes for odorant receptors and how the receptors link to the brain. |
* The 2004 [[Nobel Prize]] in [[Nobel Prize in Physiology or Medicine|Physiology or Medicine]] ([http://nobelprize.org/medicine/laureates/2004/index.html announced] 4 October 2004) was won by [[Richard Axel]] and [[Linda Buck]] for their work explaining olfaction, published first in a joint paper in 1991 that described the very large family of about one thousand genes for odorant receptors and how the receptors link to the brain. |
||
* [https://www.scribd.com/doc/32909/Sensing-or-feeling/ Answers to several questions related to senses and human feeling from curious kids] |
* [https://www.scribd.com/doc/32909/Sensing-or-feeling/ Answers to several questions related to senses and human feeling from curious kids] |
||
*[https://web.archive.org/web/20070520084243/http://www.physpharm.fmd.uwo.ca/undergrad/sensesweb/ The Physiology of the Senses tutorial] 12 animated chapters on vision, hearing, touch, balance and memory. |
* [https://web.archive.org/web/20070520084243/http://www.physpharm.fmd.uwo.ca/undergrad/sensesweb/ The Physiology of the Senses tutorial] 12 animated chapters on vision, hearing, touch, balance and memory. |
||
{{Sensory system}} |
{{Sensory system}} |
Revision as of 21:11, 6 March 2020
The physical process during which sensory systems respond to stimuli and provide data for perception is called sensation.[1] During sensation, sense organs engage in stimulus collection and transduction.[2] Sensation is often differentiated from the related and dependent concept of perception, which processes and integrates sensory information in order to give meaning to and understand detected stimuli, giving rise to subjective perceptual experience, or qualia.[3] Sensation and perception are central to, and precede, almost all aspects of cognition, behavior, and thought.[1]

In organisms, a sensory organ consists of a group of related sensory cells that respond to a specific type of physical stimulus. Via cranial and spinal nerves, the different types of sensory receptor cells (mechanoreceptors, photoreceptors, chemoreceptors, thermoreceptors) in sensory organs transduct sensory information from sensory organs towards the central nervous system, to the sensory cortices in the brain, where sensory signals are further processed and interpreted (perceived).[1][4][5] Sensory systems are often divided into external (exteroception) and internal (interoception) sensory systems, or senses.[6][7] Sensory modalities or submodalities refer to the way sensory information is encoded or transduced.[4] Multimodality integrates different senses into one unified perceptual experience. For example, information from one sense has the potential to influence how information from another is perceived.[2] Sensation and perception are studied by a variety of related fields, most notably psychophysics, neurobiology, cognitive psychology, and cognitive science.[1]
Humans have a multitude of sensory systems. Human external sensation is based on the sensory organs of the eyes, ears, skin, inner ear, nose, and mouth. The corresponding sensory systems of the visual system (sense of vision), auditory system (sense of hearing), somatosensory system (sense of touch), vestibular system (sense of balance), olfactory system (sense of smell), and gustatory system (sense of taste) contribute, respectively, to the perceptions of vision, hearing, touch, spatial orientation, smell, and taste (flavor).[2][1] Internal sensation, or interoception, detects stimuli from internal organs and tissues. A large number of internal sensory and perceptual systems exists in humans, including propioception (body position) and nociception (pain). Further internal chemoreception and osmoreception based sensory systems lead to various perceptions, such as hunger, thirst, suffocation, and nausea, or different involuntary behaviors, such as vomiting.[6][8][9]
Nonhuman animals experience sensation and perception, with varying levels of similarity to and difference from humans and other animal species. For example, mammals, in general, have a stronger sense of smell than humans. Some animal species lack one or more human sensory system analogues, some have sensory systems that are not found in humans, while others process and interpret the same sensory information in very different ways. For example, some animals are able to detect electrical[10] and magnetic fields,[11] air moisture[12], or polarized light[13], while others sense and perceive through alternative systems, such as echolocation.[14][15] Recently, it has been suggested that plants and artificial agents may be able to detect and interpret environmental information in an analogous manner to animals.[16][17][18]
Definitions
Sensory modalities
Sensory modality refers to the way that information is encoded, which is similar to the idea of transduction. The main sensory modalities can be described on the basis of how each is transduced. Listing all the different sensory modalities, which can number as many as 17, involves separating the major senses into more specific categories, or submodalities, of the larger sense. An individual sensory modality represents the sensation of a specific type of stimulus. For example, the general sensation and perception of touch, which is known as somatosensation, can be separated into light pressure, deep pressure, vibration, itch, pain, temperature, or hair movement, while the general sensation and perception of taste can be separated into submodalities of sweet, salty, sour, bitter, and umami, all of which are based on different chemicals binding to sensory neurons.[4]
Receptors
Sensory receptors are the cells or structures that detect sensations. Stimuli in the environment activate specialized receptor cells in the peripheral nervous system. During transduction, physical stimulus is converted into action potential by receptors and transmitted towards the central nervous system for processing.[5] Different types of stimuli are sensed by different types of receptor cells. Receptor cells can be classified into types on the basis of three different criteria: cell type, position, and function. Receptors can be classified structurally on the basis of cell type and their position in relation to stimuli they sense. Receptors can further be classified functionally on the basis of the transduction of stimuli, or how the mechanical stimulus, light, or chemical changed the cell membrane potential.[4]
Structural receptor types
Location
One way to classify receptors is based on their location relative to the stimuli. An exteroceptor is a receptor that is located near a stimulus of the external environment, such as the somatosensory receptors that are located in the skin. An interoceptor is one that interprets stimuli from internal organs and tissues, such as the receptors that sense the increase in blood pressure in the aorta or carotid sinus.[4]
Cell type
The cells that interpret information about the environment can be either (1) a neuron that has a free nerve ending, with dendrites embedded in tissue that would receive a sensation; (2) a neuron that has an encapsulated ending in which the sensory nerve endings are encapsulated in connective tissue that enhances their sensitivity; or (3) a specialized receptor cell, which has distinct structural components that interpret a specific type of stimulus. The pain and temperature receptors in the dermis of the skin are examples of neurons that have free nerve endings (1). Also located in the dermis of the skin are lamellated corpuscles, neurons with encapsulated nerve endings that respond to pressure and touch (2). The cells in the retina that respond to light stimuli are an example of a specialized receptor (3), a photoreceptor.[4]
A transmembrane protein receptor is a protein in the cell membrane that mediates a physiological change in a neuron, most often through the opening of ion channels or changes in the cell signaling processes. Transmembrane receptors are activated by chemicals called ligands. For example, a molecule in food can serve as a ligand for taste receptors. Other transmembrane proteins, which are not accurately called receptors, are sensitive to mechanical or thermal changes. Physical changes in these proteins increase ion flow across the membrane, and can generate an action potential or a graded potential in the sensory neurons.[4]
Functional receptor types
A third classification of receptors is by how the receptor transduces stimuli into membrane potential changes. Stimuli are of three general types. Some stimuli are ions and macromolecules that affect transmembrane receptor proteins when these chemicals diffuse across the cell membrane. Some stimuli are physical variations in the environment that affect receptor cell membrane potentials. Other stimuli include the electromagnetic radiation from visible light. For humans, the only electromagnetic energy that is perceived by our eyes is visible light. Some other organisms have receptors that humans lack, such as the heat sensors of snakes, the ultraviolet light sensors of bees, or magnetic receptors in migratory birds.[4]
Receptor cells can be further categorized on the basis of the type of stimuli they transduce. The different types of functional receptor cell types are mechanoreceptors, photoreceptors, chemoreceptors (osmoreceptor), thermoreceptors, and nociceptors. Physical stimuli, such as pressure and vibration, as well as the sensation of sound and body position (balance), are interpreted through a mechanoreceptor. Photoreceptors convert light (visible electromagnetic radiation) into signals. Chemical stimuli can be interpreted by a chemoreceptor that interprets chemical stimuli, such as an object’s taste or smell, while osmoreceptors respond to a chemical solute concentrations of body fluids. Nociception (pain) interprets the presence of tissue damage, from sensory information from mechano-, chemo-, and thermoreceptors.[19] Another physical stimulus that has its own type of receptor is temperature, which is sensed through a thermoreceptor that is either sensitive to temperatures above (heat) or below (cold) normal body temperature.[4]
Thresholds
Absolute threshold
Each sense organ (eyes or nose, for instance) requires a minimal amount of stimulation in order to detect a stimulus. This minimum amount of stimulus is called the absolute threshold.[2] The absolute threshold is defined as the minimum amount of stimulation necessary for the detection of a stimulus 50% of the time.[1] Absolute threshold is measured by using a method called signal detection. This process involves presenting stimuli of varying intensities to a subject in order to determine the level at which the subject can reliably detect stimulation in a given sense.[2]
Differential threshold
Differential threshold or just noticeable difference (JDS) is the smallest detectable difference between two stimuli, or the smallest difference in stimuli that can be judged to be different from each other.[1] Weber's Law is an empirical law that states that the difference threshold is a constant fraction of the comparison stimulus.[1] According to Weber's Law, bigger stimuli require larger differences to be noticed.[2]
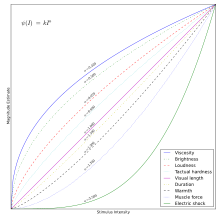
Magnitude estimation is a psychophysical method in which subjects assign perceived values of given stimuli. The relationship between stimulus intensity and perceptive intensity is described by Steven's power law.[1]
Signal detection theory
Signal detection theory quantifies the experience of the subject to the presentation of a stimulus in the presence of noise. There is internal noise and there is external noise when it comes to signal detection. The internal noise originates from static in the nervous system. For example, an individual with closed eyes in a dark room still sees something - a blotchy pattern of grey with intermittent brighter flashes -, this is internal noise. External noise is the result of noise in the environment that can interfere with the detection of the stimulus of interest. Noise is only a problem if the magnitude of the noise is large enough to interfere with signal collection. The nervous system calculates a criterion, or an internal threshold, for the detection of a signal in the presence of noise. If a signal is judged to be above the criterion, thus the signal is differentiated from the noise, the signal is sensed and perceived. Errors in signal detection can potentially lead to false positives and false negatives. The sensory criterion might be shifted based on the importance of the detecting the signal. Shifting of the criterion may influence the likelihood of false positives and false negatives.[1]
Private perceptive experience
Subjective visual and auditory experiences appear to be similar across humans subjects. The same cannot be said about taste. For example, there is a molecule called propylthiouracil (PROP) that some humans experience as bitter, some as almost tasteless, while others experience it as somewhere between tasteless and bitter. There is a genetic basis for this difference between perception given the same sensory stimulus. This subjective difference in taste perception has implications for individuals' food preferences, and consequently, health.[1]
Sensory adaptation
When a stimulus is constant and unchanging, perceptual sensory adaptation occurs. During this process, the subject becomes less sensitive to the stimulus.[2]
Fourier analysis
Biological auditory (hearing), vestibular and spatial, and visual systems (vision) appear to break down real-world complex stimuli into sine wave components, through the mathematical process called Fourier analysis. Many neurons have a strong preference for certain sine frequency components in contrast to others. The way how simpler sounds and images are encoded during sensation can provide insight into how perception of real-world objects happen.[1]
Sensory neuroscience and the biology of perception
Perception occurs when nerves that lead from the sensory organs (e.g. eye) to the brain are stimulated, even if that stimulation is unrelated to the target signal of the sensory organ. For example, in the case of the eye, it does not matter whether light or something else stimulates the optic nerve, that stimulation will results in visual perception, even if there was no visual stimulus to begin with. (To prove this point to yourself (and if you are a human), close your eyes (preferably in a dark room) and press gently on the outside corner of one eye through the eyelid. You will see a visual spot toward the inside of your visual field, near your nose.)[1]
Sensory nervous system
All stimuli received by the receptors are transduced to an action potential, which is carried along one or more afferent neurons towards a specific area (cortex) of the brain. Just as different nerves are dedicated to sensory and motors tasks, different areas of the brain (cortices) are similarly dedicated to different sensory and perceptual tasks. More complex processing is accomplished across primary cortical regions that spread beyond the primary cortices. Every nerve, sensory or motor, has its own signal transmission speed. For example, nerves in the frog's legs have a 90 ft/s (99 km/h) signal transmission speed, while sensory nerves in humans, transmit sensory information at speeds between 165 ft/s (181 km/h) and 330 ft/s (362 km/h).[1]
Multimodal perception
Perceptual experience is often multimodal. Multimodality integrates different senses into one unified perceptual experience. Information from one sense has the potential to influence how information from another is perceived.[2] Multimodal perception is qualitatively different from unimodal perception. There has been a growing body of evidence since the mid-90’s on the neural correlates of multimodal perception.[21]
Philosophy
Historical inquiries into the underlying mechanisms of sensation and perception have lead early researchers to subscribe to various philosophical interpretations of perception and the mind, including panpsychism, dualism, and materialism. The majority of modern scientists who study sensation and perception takes on a materialistic view of the mind.[1]
Human sensation
General
Absolute Threshold
Some examples of human absolute thresholds for the six external senses.[22]
Sense | Absolute threshold |
---|---|
Vision | Stars at night; Candlelight 48 km (30 mi) away on a dark and clear night |
Hearing | Ticking of a watch 6 m (20 ft) away, in an otherwise silent environment |
Vestibular | Tilt of less 30 seconds (0.0083 degrees) on a clocks face |
Touch | A wing of fly falling on the cheek from a height of 7.6 cm (3 inches) |
Taste | A teaspoon of sugar in 7.5 liters (2 gallons) of water |
Smell | A drop of perfume in a volume of the size of three rooms |
Multimodal perception
Humans respond more strongly to multimodal stimuli compared to the sum of each single modality together, an effect called the superadditive effect of multisensory integration.[2] Neurons that respond to both visual and auditory stimuli have been identified in the superior temporal sulcus.[21] Additionally, multimodal “what” and “where” pathways have been proposed for auditory and tactile stimuli.[23]
External
External receptors that respond to stimuli from outside the body are called extoreceptors.[24] Human external sensation is based on the sensory organs of the eyes, ears, skin, vestibular system, nose, and mouth, which contribute, respectively, to the sensory perceptions of vision, hearing, touch, spatial orientation, smell, and taste. Smell and taste are both responsible for identifying molecules and thus both are types of chemoreceptors. Both olfaction (smell) and gustation (taste) require the transduction of chemical stimuli into electrical potentials.[2][1]
Visual system (vision)
The visual system, or sense of sight, is based on the transduction of light stimuli received through the eyes and contributes to visual perception. The visual system detects light on photoreceptors in the retina of each eye that generates electrical nerve impulses for the perception of varying colors and brightness. There are two types of photoreceptors: rods and cones. Rods are very sensitive to light but do not distinguish colors. Cones distinguish colors but are less sensitive to dim light.[4]
At the molecular level, visual stimuli cause changes in the photopigment molecule that lead to changes in membrane potential of the photoreceptor cell. A single unit of light is called a photon, which is described in physics as a packet of energy with properties of both a particle and a wave. The energy of a photon is represented by its wavelength, with each wavelength of visible light corresponding to a particular color. Visible light is electromagnetic radiation with a wavelength between 380 and 720 nm. Wavelengths of electromagnetic radiation longer than 720 nm fall into the infrared range, whereas wavelengths shorter than 380 nm fall into the ultraviolet range. Light with a wavelength of 380 nm is blue whereas light with a wavelength of 720 nm is dark red. All other colors fall between red and blue at various points along the wavelength scale.[4]
The three types of cone opsins, being sensitive to different wavelengths of light, provide us with color vision. By comparing the activity of the three different cones, the brain can extract color information from visual stimuli. For example, a bright blue light that has a wavelength of approximately 450 nm would activate the “red” cones minimally, the “green” cones marginally, and the “blue” cones predominantly. The relative activation of the three different cones is calculated by the brain, which perceives the color as blue. However, cones cannot react to low-intensity light, and rods do not sense the color of light. Therefore, our low-light vision is—in essence—in grayscale. In other words, in a dark room, everything appears as a shade of gray. If you think that you can see colors in the dark, it is most likely because your brain knows what color something is and is relying on that memory.[4]
There is some disagreement as to whether the visual system consists of one, two, or thre submodalities. Neuroanatomists generally regard it as two submodalities, given that different receptors are responsible for the perception of color and brightness. Some argue[citation needed] that stereopsis, the perception of depth using both eyes, also constitutes a sense, but it is generally regarded as a cognitive (that is, post-sensory) function of the visual cortex of the brain where patterns and objects in images are recognized and interpreted based on previously learned information. This is called visual memory.
The inability to see is called blindness. Blindness may result from damage to the eyeball, especially to the retina, damage to the optic nerve that connects each eye to the brain, and/or from stroke (infarcts in the brain). Temporary or permanent blindness can be caused by poisons or medications. People who are blind from degradation or damage to the visual cortex, but still have functional eyes, are actually capable of some level of vision and reaction to visual stimuli but not a conscious perception; this is known as blindsight. People with blindsight are usually not aware that they are reacting to visual sources, and instead just unconsciously adapt their behavior to the stimulus.
On February 14, 2013 researchers developed a neural implant that gives rats the ability to sense infrared light which for the first time provides living creatures with new abilities, instead of simply replacing or augmenting existing abilities.[25]
Auditory system (hearing)
Hearing, or audition, is the transduction of sound waves into a neural signal that is made possible by the structures of the ear. The large, fleshy structure on the lateral aspect of the head is known as the auricle. At the end of the auditory canal is the tympanic membrane, or ear drum, which vibrates after it is struck by sound waves. The auricle, ear canal, and tympanic membrane are often referred to as the external ear. The middle ear consists of a space spanned by three small bones called the ossicles. The three ossicles are the malleus, incus, and stapes, which are Latin names that roughly translate to hammer, anvil, and stirrup. The malleus is attached to the tympanic membrane and articulates with the incus. The incus, in turn, articulates with the stapes. The stapes is then attached to the inner ear, where the sound waves will be transduced into a neural signal. The middle ear is connected to the pharynx through the Eustachian tube, which helps equilibrate air pressure across the tympanic membrane. The tube is normally closed but will pop open when the muscles of the pharynx contract during swallowing or yawning.[4]
Mechanoreceptors turn motion into electrical nerve pulses, which are located in the inner ear. Since sound is vibration, propagating through a medium such as air, the detection of these vibrations, that is the sense of the hearing, is a mechanical sense because these vibrations are mechanically conducted from the eardrum through a series of tiny bones to hair-like fibers in the inner ear, which detect mechanical motion of the fibers within a range of about 20 to 20,000 hertz,[26] with substantial variation between individuals. Hearing at high frequencies declines with an increase in age. Inability to hear is called deafness or hearing impairment. Sound can also be detected as vibrations conducted through the body by tactition. Lower frequencies that can be heard are detected this way. Some deaf people are able to determine the direction and location of vibrations picked up through the feet.[27]
Somatosensory system (touch)
Somatosensation is considered a general sense, as opposed to the special senses discussed in this section. Somatosensation is the group of sensory modalities that are associated with touch and interoception. The modalities of sometosensation include pressure, vibration, light touch, tickle, itch, temperature, pain, kinesthesia.[4] Somatosensation, also called tactition (adjectival form: tactile) is a perception resulting from activation of neural receptors, generally in the skin including hair follicles, but also in the tongue, throat, and mucosa. A variety of pressure receptors respond to variations in pressure (firm, brushing, sustained, etc.). The touch sense of itching caused by insect bites or allergies involves special itch-specific neurons in the skin and spinal cord.[28] The loss or impairment of the ability to feel anything touched is called tactile anesthesia. Paresthesia is a sensation of tingling, pricking, or numbness of the skin that may result from nerve damage and may be permanent or temporary.
Two types of somatosensory signals that are transduced by free nerve endings are pain and temperature. These two modalities use thermoreceptors and nociceptors to transduce temperature and pain stimuli, respectively. Temperature receptors are stimulated when local temperatures differ from body temperature. Some thermoreceptors are sensitive to just cold and others to just heat. Nociception is the sensation of potentially damaging stimuli. Mechanical, chemical, or thermal stimuli beyond a set threshold will elicit painful sensations. Stressed or damaged tissues release chemicals that activate receptor proteins in the nociceptors. For example, the sensation of heat associated with spicy foods involves capsaicin, the active molecule in hot peppers.[4]
Low frequency vibrations are sensed by mechanoreceptors called Merkel cells, also known as type I cutaneous mechanoreceptors. Merkel cells are located in the stratum basale of the epidermis. Deep pressure and vibration is transduced by lamellated (Pacinian) corpuscles, which are receptors with encapsulated endings found deep in the dermis, or subcutaneous tissue. Light touch is transduced by the encapsulated endings known as tactile (Meissner) corpuscles. Follicles are also wrapped in a plexus of nerve endings known as the hair follicle plexus. These nerve endings detect the movement of hair at the surface of the skin, such as when an insect may be walking along the skin. Stretching of the skin is transduced by stretch receptors known as bulbous corpuscles. Bulbous corpuscles are also known as Ruffini corpuscles, or type II cutaneous mechanoreceptors.[4]
The heat receptors are sensitive to infrared radiation and can occur in specialized organs, for instance in pit vipers. The thermoceptors in the skin are quite different from the homeostatic thermoceptors in the brain (hypothalamus), which provide feedback on internal body temperature.
Vestibular system (balance)
The vestibular sense, or sense of balance (equilibrium), is the sense that contributes to the perception of balance (equilibrium), spatial orientation, direction, or acceleration (equilibrioception). Along with audition, the inner ear is responsible for encoding information about equilibrium. A similar mechanoreceptor—a hair cell with stereocilia—senses head position, head movement, and whether our bodies are in motion. These cells are located within the vestibule of the inner ear. Head position is sensed by the utricle and saccule, whereas head movement is sensed by the semicircular canals. The neural signals generated in the vestibular ganglion are transmitted through the vestibulocochlear nerve to the brain stem and cerebellum.[4]
The semicircular canals are three ring-like extensions of the vestibule. One is oriented in the horizontal plane, whereas the other two are oriented in the vertical plane. The anterior and posterior vertical canals are oriented at approximately 45 degrees relative to the sagittal plane. The base of each semicircular canal, where it meets with the vestibule, connects to an enlarged region known as the ampulla. The ampulla contains the hair cells that respond to rotational movement, such as turning the head while saying “no.” The stereocilia of these hair cells extend into the cupula, a membrane that attaches to the top of the ampulla. As the head rotates in a plane parallel to the semicircular canal, the fluid lags, deflecting the cupula in the direction opposite to the head movement. The semicircular canals contain several ampullae, with some oriented horizontally and others oriented vertically. By comparing the relative movements of both the horizontal and vertical ampullae, the vestibular system can detect the direction of most head movements within three-dimensional (3D) space.[4]
The vestibular nerve conducts information from sensory receptors in three ampulla that sense motion of fluid in three semicircular canals caused by three-dimensional rotation of the head. The vestibular nerve also conducts information from the utricle and the saccule, which contain hair-like sensory receptors that bend under the weight of otoliths (which are small crystals of calcium carbonate) that provide the inertia needed to detect head rotation, linear acceleration, and the direction of gravitational force.
Gustatory system (taste)
The gustatory system or the sense of taste is the sensory system that is partially responsible for the perception of taste (flavor).[29] A few recognized submodalities exist within taste: sweet, salty, sour, bitter, and umami. Very recent research has suggested that there may also be a sixth taste submodality for fats, or lipids.[4] The sense of taste is often confused with the perception of flavor, which is the results of the multimodal integration of gustatory (taste) and olfactory (smell) sensations.[30]

Within the structure of the lingual papillae are taste buds that contain specialized gustatory receptor cells for the transduction of taste stimuli. These receptor cells are sensitive to the chemicals contained within foods that are ingested, and they release neurotransmitters based on the amount of the chemical in the food. Neurotransmitters from the gustatory cells can activate sensory neurons in the facial, glossopharyngeal, and vagus cranial nerves.[4]
Salty and sour taste submodalities are triggered by the cations Na+ and H+, respectively. The other taste modalities result from food molecules binding to a G protein–coupled receptor. A G protein signal transduction system ultimately leads to depolarization of the gustatory cell. The sweet taste is the sensitivity of gustatory cells to the presence of glucose (or sugar substitutes) dissolved in the saliva. Bitter taste is similar to sweet in that food molecules bind to G protein–coupled receptors. The taste known as umami is often referred to as the savory taste. Like sweet and bitter, it is based on the activation of G protein–coupled receptors by a specific molecule.[4]
Once the gustatory cells are activated by the taste molecules, they release neurotransmitters onto the dendrites of sensory neurons. These neurons are part of the facial and glossopharyngeal cranial nerves, as well as a component within the vagus nerve dedicated to the gag reflex. The facial nerve connects to taste buds in the anterior third of the tongue. The glossopharyngeal nerve connects to taste buds in the posterior two thirds of the tongue. The vagus nerve connects to taste buds in the extreme posterior of the tongue, verging on the pharynx, which are more sensitive to noxious stimuli such as bitterness.[4]
Flavor depends on odor, texture, and temperature as well as on taste. Humans receive tastes through sensory organs called taste buds, or gustatory calyculi, concentrated on the upper surface of the tongue. Other tastes such as calcium[31][32] and free fatty acids[33] may also be basic tastes but have yet to receive widespread acceptance. The inability to taste is called ageusia.
Olfactory system (smell)
Like the sense of taste, the sense of smell, or the olfactiory system, is also responsive to chemical stimuli.[4] Unlike taste, there are hundreds of olfactory receptors (388 according to one source), each binding to a particular molecular feature. Odor molecules possess a variety of features and, thus, excite specific receptors more or less strongly. This combination of excitatory signals from different receptors makes up what humans perceive as the molecule's smell.[34]
The olfactory receptor neurons are located in a small region within the superior nasal cavity. This region is referred to as the olfactory epithelium and contains bipolar sensory neurons. Each olfactory sensory neuron has dendrites that extend from the apical surface of the epithelium into the mucus lining the cavity. As airborne molecules are inhaled through the nose, they pass over the olfactory epithelial region and dissolve into the mucus. These odorant molecules bind to proteins that keep them dissolved in the mucus and help transport them to the olfactory dendrites. The odorant–protein complex binds to a receptor protein within the cell membrane of an olfactory dendrite. These receptors are G protein–coupled, and will produce a graded membrane potential in the olfactory neurons.[4]
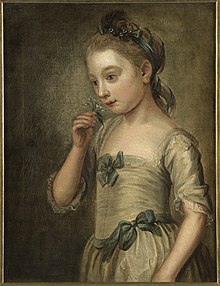
In the brain, olfaction is processed by the olfactory cortex. Olfactory receptor neurons in the nose differ from most other neurons in that they die and regenerate on a regular basis. The inability to smell is called anosmia. Some neurons in the nose are specialized to detect pheromones.[35] Loss of the sense of smell can result in food tasting bland. A person with an impaired sense of smell may require additional spice and seasoning levels for food to be tasted. Anosmia may also be related to some presentations of mild depression, because the loss of enjoyment of food may lead to a general sense of despair. The ability of olfactory neurons to replace themselves decreases with age, leading to age-related anosmia. This explains why some elderly people salt their food more than younger people do.[4]
Internal
Proprioception
Proprioception, the kinesthetic sense, provides the parietal cortex of the brain with information on the movement and relative positions of the parts of the body. Neurologists test this sense by telling patients to close their eyes and touch their own nose with the tip of a finger. Assuming proper proprioceptive function, at no time will the person lose awareness of where the hand actually is, even though it is not being detected by any of the other senses. Proprioception and touch are related in subtle ways, and their impairment results in surprising and deep deficits in perception and action.[36]
Pain
Nociception (physiological pain) signals nerve-damage or damage to tissue. The three types of pain receptors are cutaneous (skin), somatic (joints and bones), and visceral (body organs). It was previously believed that pain was simply the overloading of pressure receptors, but research in the first half of the 20th century indicated that pain is a distinct phenomenon that intertwines with all of the other senses, including touch. Pain was once considered an entirely subjective experience, but recent studies show that pain is registered in the anterior cingulate gyrus of the brain.[37] The main function of pain is to attract our attention to dangers and motivate us to avoid them. For example, humans avoid touching a sharp needle, or hot object, or extending an arm beyond a safe limit because it is dangerous, and thus hurts. Without pain, people could do many dangerous things without being aware of the dangers.
Other internal sensations and perceptions
An internal sensation and perception also known as interoception[38] is "any sense that is normally stimulated from within the body".[39] These involve numerous sensory receptors in internal organs. Interoception is thought to be atypical in clinical conditions such as alexithymia.[40] Some examples of specific receptors are:
- Hunger is governed by a set of brain structures (e.g., the hypothalamus) that are responsible for energy homeostasis.[41]
- Pulmonary stretch receptors are found in the lungs and control the respiratory rate.
- Peripheral chemoreceptors in the brain monitor the carbon dioxide and oxygen levels in the brain to give a perception of suffocation if carbon dioxide levels get too high.[42]
- The chemoreceptor trigger zone is an area of the medulla in the brain that receives inputs from blood-borne drugs or hormones, and communicates with the vomiting center.
- Chemoreceptors in the circulatory system also measure salt levels and prompt thirst if they get too high; they can also respond to high blood sugar levels in diabetics.
- Cutaneous receptors in the skin not only respond to touch, pressure, temperature and vibration, but also respond to vasodilation in the skin such as blushing.
- Stretch receptors in the gastrointestinal tract sense gas distension that may result in colic pain.
- Stimulation of sensory receptors in the esophagus result in sensations felt in the throat when swallowing, vomiting, or during acid reflux.
- Sensory receptors in pharynx mucosa, similar to touch receptors in the skin, sense foreign objects such as mucous and food that may result in a gag reflex and corresponding gagging sensation.
- Stimulation of sensory receptors in the urinary bladder and rectum may result in perceptions of fullness.
- Stimulation of stretch sensors that sense dilation of various blood vessels may result in pain, for example headache caused by vasodilation of brain arteries.
- Cardioception refers to the perception of the activity of the heart.[43][44][45][46]
- Opsins and direct DNA damage in melanocytes and keratinocytes can sense ultraviolet radiation, which plays a role in pigmentation and sunburn.
- Baroreceptors relay blood pressure information to the brain and maintain proper homeostatic blood pressure.
Nonhuman animal sensation and perception
Human analogues
Other living organisms have receptors to sense the world around them, including many of the senses listed above for humans. However, the mechanisms and capabilities vary widely.
Smell
An example of smell in non-mammals is that of sharks, which combine their keen sense of smell with timing to determine the direction of a smell. They follow the nostril that first detected the smell.[47] Insects have olfactory receptors on their antennae. Although it is unknown to the degree and magnitude which non-human animals can smell better than humans.[48]
Vomeronasal organ
Many animals (salamanders, reptiles, mammals) have a vomeronasal organ[49] that is connected with the mouth cavity. In mammals it is mainly used to detect pheromones of marked territory, trails, and sexual state. Reptiles like snakes and monitor lizards make extensive use of it as a smelling organ by transferring scent molecules to the vomeronasal organ with the tips of the forked tongue. In reptiles the vomeronasal organ is commonly referred to as Jacobsons organ. In mammals, it is often associated with a special behavior called flehmen characterized by uplifting of the lips. The organ is vestigial in humans, because associated neurons have not been found that give any sensory input in humans.[50]
Taste
Flies and butterflies have taste organs on their feet, allowing them to taste anything they land on. Catfish have taste organs across their entire bodies, and can taste anything they touch, including chemicals in the water.[51]
Vision
Cats have the ability to see in low light, which is due to muscles surrounding their irides–which contract and expand their pupils–as well as to the tapetum lucidum, a reflective membrane that optimizes the image. Pit vipers, pythons and some boas have organs that allow them to detect infrared light, such that these snakes are able to sense the body heat of their prey. The common vampire bat may also have an infrared sensor on its nose.[52] It has been found that birds and some other animals are tetrachromats and have the ability to see in the ultraviolet down to 300 nanometers. Bees and dragonflies[53] are also able to see in the ultraviolet. Mantis shrimps can perceive both polarized light and multispectral images and have twelve distinct kinds of color receptors, unlike humans which have three kinds and most mammals which have two kinds.[54]
Cephalopods have the ability to change color using chromatophores in their skin. Researchers believe that opsins in the skin can sense different wavelengths of light and help the creatures choose a coloration that camouflages them, in addition to light input from the eyes.[55] Other researchers hypothesize that cephalopod eyes in species which only have a single photoreceptor protein may use chromatic aberration to turn monochromatic vision into color vision,[56] explaining pupils shaped like the letter U, the letter W, or a dumbbell, as well as explaining the need for colorful mating displays.[57] Some cephalopods can distinguish the polarization of light.
Spatial orientation
Many invertebrates have a statocyst, which is a sensor for acceleration and orientation that works very differently from the mammalian's semi-circular canals.
Not human analogues
In addition, some animals have senses that humans do not, including the following:
Magnetoception
Magnetoception (or magnetoreception) is the ability to detect the direction one is facing based on the Earth's magnetic field. Directional awareness is most commonly observed in birds, which rely on their magnetic sense to navigate during migration.[58][58][59][permanent dead link][60][61] It has also been observed in insects such as bees. Cattle make use of magnetoception to align themselves in a north-south direction.[62] Magnetotactic bacteria build miniature magnets inside themselves and use them to determine their orientation relative to the Earth's magnetic field.[63][64] There has been some recent (tentative) research suggesting that the Rhodopsin in the human eye, which responds particularly well to blue light, can facilitate magnetoception in humans.[65]
Echolocation
Certain animals, including bats and cetaceans, have the ability to determine orientation to other objects through interpretation of reflected sound (like sonar). They most often use this to navigate through poor lighting conditions or to identify and track prey. There is currently an uncertainty whether this is simply an extremely developed post-sensory interpretation of auditory perceptions or it actually constitutes a separate sense. Resolution of the issue will require brain scans of animals while they actually perform echolocation, a task that has proven difficult in practice.
Blind people report they are able to navigate and in some cases identify an object by interpreting reflected sounds (especially their own footsteps), a phenomenon known as human echolocation.
Electroreception
Electroreception (or electroception) is the ability to detect electric fields. Several species of fish, sharks, and rays have the capacity to sense changes in electric fields in their immediate vicinity. For cartilaginous fish this occurs through a specialized organ called the Ampullae of Lorenzini. Some fish passively sense changing nearby electric fields; some generate their own weak electric fields, and sense the pattern of field potentials over their body surface; and some use these electric field generating and sensing capacities for social communication. The mechanisms by which electroceptive fish construct a spatial representation from very small differences in field potentials involve comparisons of spike latencies from different parts of the fish's body.
The only orders of mammals that are known to demonstrate electroception are the dolphin and monotreme orders. Among these mammals, the platypus[66] has the most acute sense of electroception.
A dolphin can detect electric fields in water using electroreceptors in vibrissal crypts arrayed in pairs on its snout and which evolved from whisker motion sensors.[67] These electroreceptors can detect electric fields as weak as 4.6 microvolts per centimeter, such as those generated by contracting muscles and pumping gills of potential prey. This permits the dolphin to locate prey from the seafloor where sediment limits visibility and echolocation.
Spiders have been shown to detect electric fields to determine a suitable time to extend web for 'ballooning'.[68]
Body modification enthusiasts have experimented with magnetic implants to attempt to replicate this sense.[69] However, in general humans (and it is presumed other mammals) can detect electric fields only indirectly by detecting the effect they have on hairs. An electrically charged balloon, for instance, will exert a force on human arm hairs, which can be felt through tactition and identified as coming from a static charge (and not from wind or the like). This is not electroreception, as it is a post-sensory cognitive action.
Hygroreception
Hygroreception is the ability to detect changes in the moisture content of the environment.[12][70]
Infrared sensing
The ability to sense infrared thermal radiation evolved independently in various families of snakes. Essentially, it allows these reptiles to "see" radiant heat at wavelengths between 5 and 30 μm to a degree of accuracy such that a blind rattlesnake can target vulnerable body parts of the prey at which it strikes.[71] It was previously thought that the organs evolved primarily as prey detectors, but it is now believed that it may also be used in thermoregulatory decision making.[72] The facial pit underwent parallel evolution in pitvipers and some boas and pythons, having evolved once in pitvipers and multiple times in boas and pythons.[73] The electrophysiology of the structure is similar between the two lineages, but they differ in gross structural anatomy. Most superficially, pitvipers possess one large pit organ on either side of the head, between the eye and the nostril (Loreal pit), while boas and pythons have three or more comparatively smaller pits lining the upper and sometimes the lower lip, in or between the scales. Those of the pitvipers are the more advanced, having a suspended sensory membrane as opposed to a simple pit structure. Within the family Viperidae, the pit organ is seen only in the subfamily Crotalinae: the pitvipers. The organ is used extensively to detect and target endothermic prey such as rodents and birds, and it was previously assumed that the organ evolved specifically for that purpose. However, recent evidence shows that the pit organ may also be used for thermoregulation. According to Krochmal et al., pitvipers can use their pits for thermoregulatory decision-making while true vipers (vipers who do not contain heat-sensing pits) cannot.
In spite of its detection of IR light, the pits' IR detection mechanism is not similar to photoreceptors – while photoreceptors detect light via photochemical reactions, the protein in the pits of snakes is in fact a temperature-sensitive ion channel. It senses infrared signals through a mechanism involving warming of the pit organ, rather than a chemical reaction to light.[74] This is consistent with the thin pit membrane, which allows incoming IR radiation to quickly and precisely warm a given ion channel and trigger a nerve impulse, as well as vascularize the pit membrane in order to rapidly cool the ion channel back to its original "resting" or "inactive" temperature.[74]
Other
Pressure detection uses the organ of Weber, a system consisting of three appendages of vertebrae transferring changes in shape of the gas bladder to the middle ear. It can be used to regulate the buoyancy of the fish. Fish like the weather fish and other loaches are also known to respond to low pressure areas but they lack a swim bladder.
Current detection is a detection system of water currents, consisting mostly of vortices, found in the lateral line of fish and aquatic forms of amphibians. The lateral line is also sensitive to low-frequency vibrations. The mechanoreceptors are hair cells, the same mechanoreceptors for vestibular sense and hearing. It is used primarily for navigation, hunting, and schooling. The receptors of the electrical sense are modified hair cells of the lateral line system.
Polarized light direction/detection is used by bees to orient themselves, especially on cloudy days. Cuttlefish, some beetles, and mantis shrimp can also perceive the polarization of light. Most sighted humans can in fact learn to roughly detect large areas of polarization by an effect called Haidinger's brush, however this is considered an entoptic phenomenon rather than a separate sense.
Slit sensillae of spiders detect mechanical strain in the exoskeleton, providing information on force and vibrations.
Plant sensation
By using a variety of sense receptors, plants sense light, temperature, humidity, chemical substances, chemical gradients, reorientation, magnetic fields, infections, tissue damage and mechanical pressure. The absence of a nervous system notwithstanding, plants interpret and respond to these stimuli by a variety of hormonal and cell-to-cell communication pathways that result in movement, morphological changes and physiological state alterations at the organism level, that is, result in plant behavior. Such physiological and cognitive functions are generally not believed to give rise to mental phenomena or qualia, however, as these are typically considered the product of nervous system activity. The emergence of mental phenomena from the activity of systems functionally or computationally analogous to that of nervous systems is, however, a hypothetical possibility explored by some schools of thought in the philosophy of mind field, such as functionalism and computationalism.
However, plants could perceive the world around them,[16] and might be able to emit airborne sounds similar to "screaming" when stressed. Those noises could not be detectable by human ears, but organisms with a hearing range that can hear ultrasonic frequencies—like mice, bats or perhaps other plants—could hear the plants' cries from as far as 15 feet (4.6 m) away.[75]
Artificial sensation and perception
Machine perception is the capability of a computer system to interpret data in a manner that is similar to the way humans use their senses to relate to the world around them.[17][18][76] Computers take in and respond to their environment through attached hardware. Until recently, input was limited to a keyboard, or a mouse, but advances in technology, both in hardware and software, have allowed computers to take in sensory input in a way similar to humans.[17][18]
Culture
![]() | This section may require cleanup to meet Wikipedia's quality standards. The specific problem is: This section may contain original research. Additional citations are needed. (2020-03-03) |



In the time of William Shakespeare, there were commonly reckoned to be five wits or five senses.[78] At that time, the words "sense" and "wit" were synonyms,[78] so the senses were known as the five outward wits.[79][80] This traditional concept of five senses is common today.
The traditional five senses are enumerated as the "five material faculties" (pañcannaṃ indriyānaṃ avakanti) in Hindu literature. They appear in allegorical representation as early as in the Katha Upanishad (roughly 6th century BC), as five horses drawing the "chariot" of the body, guided by the mind as "chariot driver".
Depictions of the five traditional senses as allegory became a popular subject for seventeenth-century artists, especially among Dutch and Flemish Baroque painters. A typical example is Gérard de Lairesse's Allegory of the Five Senses (1668), in which each of the figures in the main group alludes to a sense: Sight is the reclining boy with a convex mirror, hearing is the cupid-like boy with a triangle, smell is represented by the girl with flowers, taste is represented by the woman with the fruit, and touch is represented by the woman holding the bird.
In Buddhist philosophy, Ayatana or "sense-base" includes the mind as a sense organ, in addition to the traditional five. This addition to the commonly acknowledged senses may arise from the psychological orientation involved in Buddhist thought and practice. The mind considered by itself is seen as the principal gateway to a different spectrum of phenomena that differ from the physical sense data. This way of viewing the human sense system indicates the importance of internal sources of sensation and perception that complements our experience of the external world.[citation needed]
See also
- Aesthesis
- Apperception
- Attention
- Āyatana (sense bases in Theravada Buddhism)
- Chemesthesis
- Empiricism
- Extrasensory Perception
- Entoptic phenomenon
- Increased sensitivity:
- Illusions
- Intuition
- Multisensory integration
- Phantom limb
- Remote sensing
- Saḷāyatana and Ayatana (the six senses as a concept in Buddhism)
- Sensation and perception psychology
- Sense of direction
- Sensitivity (human)
- Sensorium
- Sensory processing disorder
- Sensus divinitatis
- Synesthesia (Ideasthesia)
References
- ^ a b c d e f g h i j k l m n o p q Wolfe, Jeremy; Kluender, Keith; Levi, Dennis. Sensation & perception (3rd ed.). Sinauer Associates. p. 7. ISBN 978-0-87893-572-7.
- ^ a b c d e f g h i j Privitera, A. J. (2020). Sensation and perception. In R. Biswas-Diener & E. Diener (Eds), Noba textbook series: Psychology. Champaign, IL: DEF publishers. Retrieved from http://noba.to/xgk3ajhy
- ^ Schacter, Daniel (2011). Psychology. Worth Publishers.
- ^ a b c d e f g h i j k l m n o p q r s t u v w x y z Anatomy and Physiology. Rice University (OpenStax). 2016-02-26.
- ^ a b Molecular cell biology. Lodish, Harvey F. (4th ed.). New York: W.H. Freeman. 2000. ISBN 0716731363. OCLC 41266312.
{{cite book}}
: CS1 maint: others (link) - ^ a b Campbell, Neil A. (2017). Biology. Pearson Education UK. ISBN 978-1-292-17044-2. OCLC 1017000156.
- ^ Tsakiris, Manos, editor. Preester, Helena de, editor. The interoceptive mind : from homeostasis to awareness. ISBN 0-19-881193-4. OCLC 1036733582.
{{cite book}}
:|last=
has generic name (help)CS1 maint: multiple names: authors list (link) - ^ Tsakiris, Manos, editor. Preester, Helena de, editor. The interoceptive mind : from homeostasis to awareness. ISBN 0-19-881193-4. OCLC 1036733582.
{{cite book}}
:|last=
has generic name (help)CS1 maint: multiple names: authors list (link) - ^ Khalsa, Sahib S.; Lapidus, Rachel C. (2016-07-25). "Can Interoception Improve the Pragmatic Search for Biomarkers in Psychiatry?". Frontiers in Psychiatry. 7. doi:10.3389/fpsyt.2016.00121. ISSN 1664-0640.
{{cite journal}}
: CS1 maint: unflagged free DOI (link) - ^ Kalmijn, A.J. (1988). "Detection of Weak Electric Fields". In Atema, Jelle; Fay, Richard R.; Popper, Arthur N.; Tavolga, William N. (eds.). Sensory Biology of Aquatic Animals. International Conference on the Sensory Biology of Aquatic Animals. Springer Nature Switzerland AG. doi:10.1007/978-1-4612-3714-3. ISBN 978-1-4612-8317-1.
- ^ Walker, Michael M.; Dennis, Todd E.; Kirschvink, Joseph L. (December 2002). "The magnetic sense and its use in long-distance navigation by animals". Current Opinion in Neurobiology. 12 (6): 735–744. doi:10.1016/S0959-4388(02)00389-6. PMID 12490267.
- ^ a b Enjin A, Zaharieva EE, Frank DD, Mansourian S, Suh GS, Gallio M, Stensmyr MC (May 2016). "Humidity Sensing in Drosophila". Current Biology. 26 (10): 1352–8. Bibcode:1996CBio....6.1213A. doi:10.1016/j.cub.2016.03.049. PMC 5305172. PMID 27161501.
- ^ Cronin, T.W. (2010), "Polarized-Light Vision in Land and Aquatic Animals", Encyclopedia of the Eye, Elsevier, pp. 461–468, ISBN 978-0-12-374203-2, retrieved 2020-03-04
- ^ Fenton, M. Brock. editor. Grinnell, Alan D. editor. Popper, Arthur N. editor. Fay, Richard R. editor. Bat Bioacoustics. ISBN 1-4939-3527-5. OCLC 1127113751.
{{cite book}}
:|last=
has generic name (help)CS1 maint: multiple names: authors list (link) - ^ Kyhn, L. A.; Jensen, F. H.; Beedholm, K.; Tougaard, J.; Hansen, M.; Madsen, P. T. (2010-05-14). "Echolocation in sympatric Peale's dolphins (Lagenorhynchus australis) and Commerson's dolphins (Cephalorhynchus commersonii) producing narrow-band high-frequency clicks". Journal of Experimental Biology. 213 (11): 1940–1949. doi:10.1242/jeb.042440. ISSN 0022-0949.
- ^ a b "Earth - Plants can see, hear and smell – and respond". BBC. 10 January 2017.
- ^ a b c Les, Zbigniew; Les, Magdalena (2019-08-02), "Machine Perception—Machine Perception MU", Machine Understanding, Springer International Publishing, pp. 9–44, ISBN 978-3-030-24069-1, retrieved 2020-03-04
- ^ a b c Serov, Alexander (2013-01-27). Subjective Reality and Strong Artificial Intelligence. OCLC 1106181879.
- ^ Principles of neural science. Kandel, Eric R., Schwartz, James H. (James Harris), 1932-2006., Jessell, Thomas M. (3rd ed ed.). Norwalk, Conn.: Appleton & Lange. 1991. ISBN 0-8385-8034-3. OCLC 27216558.
{{cite book}}
:|edition=
has extra text (help)CS1 maint: others (link) - ^ Small DM, Green BG. A Proposed Model of a Flavor Modality. In: Murray MM, Wallace MT, editors. The Neural Bases of Multisensory Processes. Boca Raton (FL): CRC Press/Taylor & Francis; 2012. Chapter 36. Available from: https://www.ncbi.nlm.nih.gov/books/NBK92876/
- ^ a b Calvert, G.A., Hansen, P.C., Iversen, S.D. and Brammer, M.J., 2001. Detection of audio-visual integration sites in humans by application of electrophysiological criteria to the BOLD effect. Neuroimage, 14(2), pp.427-438.
- ^ Galanter, E. (1962). "Direct measurement of utility and subjective probability". American Journal of Psychology. 75: 208–220.
- ^ Renier, L.A., Anurova, I., De Volder, A.G., Carlson, S., VanMeter, J. and Rauschecker, J.P., 2009. Multisensory integration of sounds and vibrotactile stimuli in processing streams for “what” and “where”. Journal of Neuroscience, 29(35), pp.10950-10960.
- ^ Campbell, Neil A. (1946-2004). (cop. 2005). Biology. Pearson. ISBN 0-321-26984-5. OCLC 904492777.
{{cite book}}
: Check date values in:|date=
(help)CS1 maint: numeric names: authors list (link) - ^ "Implant gives rats sixth sense for infrared light". Wired UK. 14 February 2013. Retrieved 14 February 2013.
- ^ "Frequency Range of Human Hearing, Physics Factbook by Glenn Elert (ed)". Hypertextbook.com. Retrieved 2014-04-05.
- ^ "Deaf Culture and Communication: A Basic Guide" (PDF). Victorian Deaf Society. 2010.
- ^ Sun YG, Zhao ZQ, Meng XL, Yin J, Liu XY, Chen ZF (September 2009). "Cellular basis of itch sensation". Science. 325 (5947): 1531–4. Bibcode:2009Sci...325.1531S. doi:10.1126/science.1174868. PMC 2786498. PMID 19661382.
- ^ Trivedi, Bijal P. (2012-06). "Gustatory system: The finer points of taste". Nature. 486 (7403): S2–S3. doi:10.1038/486s2a. ISSN 0028-0836.
{{cite journal}}
: Check date values in:|date=
(help) - ^ Murray, M. M. (Micah M.), editor. Wallace, Mark T., editor. The neural bases of multisensory processes. ISBN 978-1-4398-1219-8. OCLC 759160178.
{{cite book}}
:|last=
has generic name (help)CS1 maint: multiple names: authors list (link) - ^ Tordoff MG (August 2008). "Gene discovery and the genetic basis of calcium consumption". Physiology & Behavior. 94 (5): 649–59. doi:10.1016/j.physbeh.2008.04.004. PMC 2574908. PMID 18499198.
- ^ "That Tastes ... Sweet? Sour? No, It's Definitely Calcium!". Sciencedaily.
- ^ Mattes RD (2009). "Is there a fatty acid taste?". Annual Review of Nutrition. 29: 305–27. doi:10.1146/annurev-nutr-080508-141108. PMC 2843518. PMID 19400700.
- ^ "A Sense of Smell: Olfactory Receptors". Sandwalk. 2007-01-09.
- ^ "The Surprising Impact of Taste and Smell". LiveScience.
- ^ "The Importance of the Sense of Touch in Virtual and Real Environments" (PDF). International Society for Haptics.
- ^ "Functional MR imaging of regional brain activation associated with the affective experience of pain". AJR. American Journal of Roentgenology. 177 (5): 1205–10. November 2001. doi:10.2214/ajr.177.5.1771205. PMID 11641204.
{{cite journal}}
: Cite uses deprecated parameter|authors=
(help) - ^ Craig AD (August 2003). "Interoception: the sense of the physiological condition of the body". Current Opinion in Neurobiology. 13 (4): 500–5. doi:10.1016/S0959-4388(03)00090-4. PMID 12965300.
- ^ Dunn BD, Galton HC, Morgan R, Evans D, Oliver C, Meyer M, Cusack R, Lawrence AD, Dalgleish T (December 2010). "Listening to your heart. How interoception shapes emotion experience and intuitive decision making". Psychological Science. 21 (12): 1835–44. doi:10.1177/0956797610389191. PMID 21106893.
- ^ Shah P, Hall R, Catmur C, Bird G (August 2016). "Alexithymia, not autism, is associated with impaired interoception". Cortex; A Journal Devoted to the Study of the Nervous System and Behavior. 81: 215–20. doi:10.1016/j.cortex.2016.03.021. PMC 4962768. PMID 27253723.
- ^ Farr OM, Li CS, Mantzoros CS (May 2016). "Central nervous system regulation of eating: Insights from human brain imaging". Metabolism. 65 (5): 699–713. doi:10.1016/j.metabol.2016.02.002. PMC 4834455. PMID 27085777.
- ^ "How Your Lungs Work". HowStuffWorks. 2000-10-06.
- ^ Garfinkel SN, Seth AK, Barrett AB, Suzuki K, Critchley HD (January 2015). "Knowing your own heart: distinguishing interoceptive accuracy from interoceptive awareness". Biological Psychology. 104: 65–74. doi:10.1016/j.biopsycho.2014.11.004. PMID 25451381.
- ^ Schandry R (July 1981). "Heart beat perception and emotional experience". Psychophysiology. 18 (4): 483–8. doi:10.1111/j.1469-8986.1981.tb02486.x. PMID 7267933.
- ^ Kleckner IR, Wormwood JB, Simmons WK, Barrett LF, Quigley KS (November 2015). "Methodological recommendations for a heartbeat detection-based measure of interoceptive sensitivity". Psychophysiology. 52 (11): 1432–40. doi:10.1111/psyp.12503. PMC 4821012. PMID 26265009.
- ^ Whitehead WE, Drescher VM, Heiman P, Blackwell B (December 1977). "Relation of heart rate control to heartbeat perception". Biofeedback and Self-Regulation. 2 (4): 317–92. doi:10.1007/BF00998623. PMID 612350.
- ^ Gardiner JM, Atema J (July 2010). "The function of bilateral odor arrival time differences in olfactory orientation of sharks". Current Biology. 20 (13): 1187–91. Bibcode:1996CBio....6.1213A. doi:10.1016/j.cub.2010.04.053. PMID 20541411.
- ^ Devlin, Hannah (2017-05-11). "Not to be sniffed at: human sense of smell rivals that of dogs, says study". The Guardian. ISSN 0261-3077. Retrieved 2019-04-10.
{{cite news}}
: Unknown parameter|name-list-format=
ignored (|name-list-style=
suggested) (help) - ^ Takami S (August 2002). "Recent progress in the neurobiology of the vomeronasal organ". Microscopy Research and Technique. 58 (3): 228–50. doi:10.1002/jemt.10094. PMID 12203701.
- ^ Frasnelli J, Lundström JN, Boyle JA, Katsarkas A, Jones-Gotman M (March 2011). "The vomeronasal organ is not involved in the perception of endogenous odors". Human Brain Mapping. 32 (3): 450–60. doi:10.1002/hbm.21035. PMC 3607301. PMID 20578170.
- ^ Atema, Jelle (1980) "Chemical senses, chemical signals, and feeding behavior in fishes" p. 57–101. In: Bardach, JE Fish behavior and its use in the capture and culture of fishes', The WorldFish Center, ISBN 978-971-02-0003-0.
- ^ "The illustrated story of the Vampire bat". Retrieved 2007-05-25.
- ^ van Kleef J, Berry R, Stange G (March 2008). "Directional selectivity in the simple eye of an insect". The Journal of Neuroscience. 28 (11): 2845–55. doi:10.1523/JNEUROSCI.5556-07.2008. PMC 6670670. PMID 18337415.
- ^ Marshall J, Oberwinkler J (October 1999). "The colourful world of the mantis shrimp". Nature. 401 (6756): 873–4. Bibcode:1999Natur.401..873M. doi:10.1038/44751. PMID 10553902.
- ^ Octopus vision, it's in the eye (or skin) of the beholder
- ^ Study proposes explanation for how cephalopods see color, despite black and white vision
- ^ Odd pupils let ‘colorblind’ octopuses see colors
- ^ a b "The Magnetic Sense of Animals". Theoretical and Computational Biophysics Group.
- ^ "Built-in GPS in birds in tune with Earth's magnetic field". Baylor College of Medicine.
- ^ Wu LQ, Dickman JD (May 2012). "Neural correlates of a magnetic sense". Science. 336 (6084): 1054–7. Bibcode:2012Sci...336.1054W. doi:10.1126/science.1216567. PMID 22539554.
- ^ Cressey, Daniel (2012). "Pigeons may 'hear' magnetic fields". Nature. doi:10.1038/nature.2012.10540. ISSN 1744-7933.
{{cite journal}}
: Unknown parameter|name-list-format=
ignored (|name-list-style=
suggested) (help) - ^ "Cattle shown to align north-south". BBC News - Science/Nature.
- ^ Blakemore R (October 1975). "Magnetotactic bacteria". Science. 190 (4212): 377–9. Bibcode:1975Sci...190..377B. doi:10.1126/science.170679. PMID 170679.
- ^ Urban JE (November 2000). "Adverse effects of microgravity on the magnetotactic bacterium Magnetospirillum magnetotacticum". Acta Astronautica. 47 (10): 775–80. Bibcode:2000AcAau..47..775U. doi:10.1016/S0094-5765(00)00120-X. PMID 11543576.
- ^ Chae KS, Oh IT, Lee SH, Kim SC (2019-02-14). "Blue light-dependent human magnetoreception in geomagnetic food orientation". PLOS ONE. 14 (2): e0211826. doi:10.1371/journal.pone.0211826. PMC 6375564. PMID 30763322.
{{cite journal}}
: CS1 maint: unflagged free DOI (link) - ^ "Electroreceptive Mechanisms in the Platypus". Archived from the original on 1999-02-09.
- ^ Drake, Nadia (2011). "Life: Dolphin can sense electric fields: Ability may help species track prey in murky waters". Science News. 180 (5): 12. doi:10.1002/scin.5591800512.
{{cite journal}}
: Unknown parameter|name-list-format=
ignored (|name-list-style=
suggested) (help) - ^ Morley, Erica (July 5, 2018). "Electric Fields Elicit Ballooning in Spiders". Current Biology. 28 (14).
- ^ "Implant gives man the sense of "magnetic vision"". Retrieved 2011-04-23.
- ^ Tichy H, Kallina W (2013-01-16). "The evaporative function of cockroach hygroreceptors". PLOS ONE. 8 (1): e53998. Bibcode:2013PLoSO...853998T. doi:10.1371/journal.pone.0053998. PMC 3546976. PMID 23342058.
{{cite journal}}
: CS1 maint: unflagged free DOI (link) - ^ (Kardong & Mackessy 1991)
- ^ (Krochmal et al. 2004)
- ^ (Pough et al. 1992)
- ^ a b (Gracheva et al. 2010)
- ^ I. Khait; O. Lewin-Epstein; R. Sharon; K. Saban; R. Perelman; A. Boonman; Y. Yovel; L. Hadany (2 December 2019). "Plants emit informative airborne sounds under stress" (PDF). bioRxiv. doi:10.1101/507590.
{{cite journal}}
: Cite journal requires|journal=
(help) - ^ "Machine Perception & Cognitive Robotics Laboratory". www.ccs.fau.edu. Retrieved 2016-06-18.
- ^ "Allegory of the Five Senses". The Walters Art Museum.
- ^ a b Horace Howard Furness (1880). "King Lear". Shakespeare. Vol. 5 (7th ed.). Philadelphia: J.B. Lippincott Co. p. 187. OCLC 1932507.
- ^ "wit". The Merriam-Webster new book of word histories. Merriam-Webster. 1991. pp. 508. ISBN 978-0-87779-603-9. OCLC 24246335.
- ^ Clive Staples Lewis (1990). "Sense". Studies in Words (2nd (republished) ed.). Cambridge University Press. p. 147. ISBN 978-0-521-39831-2. OCLC 489987083.
External links



- The 2004 Nobel Prize in Physiology or Medicine (announced 4 October 2004) was won by Richard Axel and Linda Buck for their work explaining olfaction, published first in a joint paper in 1991 that described the very large family of about one thousand genes for odorant receptors and how the receptors link to the brain.
- Answers to several questions related to senses and human feeling from curious kids
- The Physiology of the Senses tutorial 12 animated chapters on vision, hearing, touch, balance and memory.