Evaluation of the anti-tumor activity of histone deacetylase inhibitors (HDAC6) on human pancreatic adenocarcinoma (PANC-1) cell line using a zebrafish xenograft model
Highlight box
Key findings
• Using a zebrafish xenograft model, the study evaluated histone deacetylase 6 (HDAC6)-I and its analog on a human pancreatic adenocarcinoma (PANC-1) cell line.
What is known and what is new?
• The human PANC-1 was implanted in peri-vitelline space of 48-hour zebrafish embryos with Sirt6 inhibitor, Sirt6 analog, and without any of these two as control.
What is the implication, and what should change now?
• The findings of this study suggest that the HDAC6-I and its analog have the potency to regress tumor size and reduce the number of cells undergoing metastasis. Research should be conducted on these entities in order to use them as therapeutic agents.
Introduction
Cancer has emerged as one of the leading causes of death worldwide, accounting for nearly 10 million deaths in 2020, or nearly one in six deaths (1,2). Among various cancers, pancreatic ductal adenocarcinoma (PDAC) is considered one of the most aggressive and lethal malignancies worldwide, with a median survival time of fewer than 18 months (3,4). It remains undiagnosed at the initial stage in most cases, and mostly at the time of diagnosis, the tumor is advanced locally or has metastasized distantly. Therefore, a radical surgical resection for a curative treatment option can be performed in <20% of cases only as most patients have an advanced stage of the disease at the diagnosis.
In pancreatic cancers, the signaling pathways governing organogenesis are often deregulated in tumors (5,6). Tumorigenesis, wherein normal cells are transformed into malignant cells, is a result of major biological changes occurring in the neoplastic cells, which is called the hallmark of cancer (7). Besides mutations in the key driver genes, deregulation of the above epigenetic mechanisms, such as histone acetylation and deacetylation are also considered to be an underlying cause of cancer development (8). The balance of acetylation and deacetylation of lysine residues is critical in sustaining body homeostasis, and any disruption of this balance can lead to cancer as well as many developmental disorders in humans (9). Pancreatic cancer is the seventh leading cause of cancer-related deaths worldwide. The incidence of pancreatic cancer varies across regions and populations. Globally its incidence is 5.5 per 100,000 in men and 4.0 per 100,000 in women (3,4).
Histone deacetylase 6 (HDAC6; Sirt6) is a member of the HDAC family that participates in histone acetylation and deacetylation and also targets several non-histone substrates, such as α-tubulin, cortactin, and heat shock protein 90 (HSP90), to regulate cell proliferation, metastasis, invasion, and mitosis in tumors (10,11). Furthermore, it also forms complexes with various other proteins to regulate both pathological and physiological processes.
HDAC6 was found to be overexpressed in pancreatic cancer cells as compared to normal cells and adjacent cells in various studies (12). This upregulation might indicate their involvement in cancer proliferation. However, further studies revealed that HDAC6 is only associated with pancreatic cancer cell motility rather than its proliferation (12). HDAC also upregulates host immune responses. HDAC6, in particular, modulates the acetylation step of non-histone proteins involved in tumor processes of migration, metastasis, stress pathways, and angiogenesis (13).
Over time, researchers are trying to develop HDAC6-specific inhibitors to control the notorious disease (13). HDAC6-specific inhibitors demonstrated apoptotic activities other than modulating the acetylation step. These compounds result in cell cycle arrest in pancreatic cancer at G1 or G2 phases, they also induce apoptosis in pancreatic cancer cells. Their combination with gemcitabine (DNA-damage inducing agent) can prove to be an effective pancreatic cancer treatment (14). Studies on HDAC inhibitors have shown their strong anticancer activities and tumor specificities owing to their apoptotic effect, arrest of cell cycle, and angiogenesis inhibition (13-15). Towards this end, we aim to elucidate the role of histone deacetylase inhibitors in pancreatic cancer tumor regression and its ability to inhibit metastasis.
We wish to employ zebrafish to accomplish our goal. Zebrafish have emerged as one powerful genetic model to study the developments in vertebrates. It has been used excessively to understand the pathophysiology of diseases in humans, genetic dissection of the mechanisms underlying vertebrate organogenesis, including the formation of the pancreas, and unravel the genetic alterations associated with the progressive transformation of the pancreatic duct cell into invasive carcinoma (16,17). The ongoing work on the zebrafish model is likely to complement research carried out using other vertebrate model organisms and may help in identifying markers that define the early stages of pancreatic tumorigenesis as well as potential targets for therapy.
The use of this zebrafish has several advantages over other animal models. The small size of zebrafish, high breeding ability, ability to become sexually mature within 2–3 months, and high fecundity of female zebrafish allow us to maintain large numbers of these fish in a small area. Zebrafish embryos are transparent, thus allowing researchers to study embryogenesis and organogenesis in these fish with ease. Moreover, it also facilitates the performance of lineage tracing, green fluorescent protein (GFP) analysis, and experimentation involving cell transplantation (18).
Zebrafish is emerging as an exciting model to study different human tumor types. Due to genetic homology, physiology, and developmental similarity, zebrafish have increasingly become a desirable tool for studying the development and modelling of human disease, notably studying the initiation, progression, and also metastasis of cancer (18,19).
Furthermore, the transparency of zebrafish embryos and ease of genetic tractability due to ongoing developments in the field of visualization and genetic interference technologies are making zebrafish models even more practical to be used for diagnosis and further elucidating the pathophysiology of cancer.
Zebrafish and its embryos have been widely used in pancreatic cancer research. As stated above, pancreas organogenesis in zebrafish embryos is similar to the human pancreas, especially in terms of physiologically and morphologically. The development of the pancreas in zebrafish is completed in three stages viz gastrulation or early stage, somitogenesis or mid-stage, and larva or end-stage. At the late blastula stage, marginal cells of the embryo give rise to endoderm (4 hours post-fertilization, hpf). In the dorsal marginal area, pancreas progenitors are present, cells closer to the dorsal zone form the dorsal pancreatic bud, and those which are away from the ventral pancreatic bud. During this early stage, the hypoblast layer is formed by the involution process of the endoderm and mesoderm. At 8 hpf, definitive endoderm is formed (20). At 10 hpf, progenitors arrange at the equator (21) where inductive signals (RA) signals coming from mesoderm play a significant role in the pancreatic specification of cells (22). At this stage, all three germ layers are at the dorsal midline (23). Primary islets are formed by converging insulin cells, endodermal sheets, and pdx1+ cells at 18 hpf. Somitogenesis is completed at about 24 hpf with the formation of dorsal pancreatic buds. At about 32 hpf ventral bud appears, and the mesoderm layer between 26–29 hpf is responsible for this (24,25). Finally, at about 48 hpf or 2 days both the buds fuse morphogenetically to give rise to a mature pancreas where primary islets sit at the core of the mature pancreas (24). Therefore, using the day 2 embryo of zebrafish can prove to be significantly important when it comes to studying pancreatic diseases because it has matured pancreas with similar features to that of the human pancreas.
Thus, to achieve our aim of deciphering the role of histone deacetylase inhibitors in pancreatic cancer tumor regression and its ability to inhibit metastasis, we chose the zebrafish model. I present this article in accordance with the STROBE reporting checklist (available at https://pcm.amegroups.com/article/view/10.21037/pcm-23-8/rc).
Methods
Cell culturing
The human pancreatic adenocarcinoma (PANC-1) cell lines were obtained from ATTC (Virginia, USA) and were cultured in RPMI 1640 medium GIBCO (Montana, USA) supplemented with 10% Fetal Calf Serum, 1% L-glutamine, 1% penicillin-streptomycin. They were grown in 75 cm3 cell culturing flasks at 37 ℃ in a humidified atmosphere containing 5% CO2.
Cell staining
The cells, when they reached a confluency of about 70–80%, were processed for staining with fluorescent Dil dye. The medium from culturing flask was decanted, and cells were washed several times by adding 3 mL PBS (phosphate-buffered saline) and gentle shaking. After the final wash, PBS was discarded. Dil stock solution, 3 µL was added to a 10-mL pre-warmed PBS; this solution of Dil-PBS (10 mL) was added to the culture flask and incubated at 37 ℃ for 30 minutes. After 30 minutes, the content of the culture flask having the detached cells and staining solution was transferred to a 15-mL falcon tube and centrifuged for 5 minutes at 400 g. The supernatant was removed, and cells were washed twice by adding PBS and centrifuged for 5 minutes at 400 g each time. Lastly, the cell pellets were washed with 1 mL medium. The cells were then transferred to an Eppendorf tube and resuspended to be used for further experiments.
Preparation of the embryo
Adult Fli1:EGFP transgenic zebrafish (zebrafish facility Linkoping University) were used for this experiment and were handled in strict compliance with local animal welfare regulations. Fish were kept in a breeding tank at a mean temperature of 28 ℃ with a 14:10 hr light to dark cycle. Zebrafish fertilized eggs were obtained by natural mating between four females and two males overnight, and after 16 hours the eggs were harvested and placed in a dish with E3 medium (containing 0.286 g NaCl, 0.048 g CaCl2, 0.081 g MgSO4 and 0.0126 g KCl per liter, pH7.2) supplemented with 0.2 mM 1-Phenyl-2-thiourea (PTU) solution. PTU prevents the pigmentation of the embryo, making the zebrafish transparent facilitating observance of the injected cells by microscopy.
The 48-hour-old zebrafish embryos were manually decoronated (hatched), transferred on a 2% agarose gel, and anesthetized with 0.04% tricaine solution.
Using a micropipette puller, a microcapillary was inserted into two needles. The needles were then filled with the cell’s suspension and gently injected into the peri-vitelline space (PVS) of embryos.
Immediately after injection (day 0), the embryos were viewed under the microscope to exclude the embryos which were accidentally injected into the yolk and to take pictures of the embryo at day 0. Correctly injected embryos were then transferred to a 24-well plate filled with PTU solution supplemented with the Sirt6 inhibitor or Sirt6 analogue or vehicle (Control) and incubated 36 ℃ for 3 days. After 3 days images were captured using a DS-2 camera on a Nikon SMZ1500 fluorescence microscope under the red fluorescent light.
Quantification of tumor cells
Tumor size and total area of tumor were measured using the ImageJ software 1.46r (National Institute of Health, Bethesda, Maryland, USA). The brightness and contrast were adjusted using the menu image-adjust-brightness and contrast, and the threshold was adjusted using the menu image-adjust threshold. The total area measured within the required area was obtained by pressing the menu-analyse-analyse particles function, then the number of the total area of the tumor was recorded for all the groups and normalized. For the metastasis study, the number of cells that migrated from the PVS to the tail after three days of implantation in both the Control and treatment groups was counted.
Preparation of anticancer drugs
Sirt6 inhibitors and Sirt6 analog (obtained from Stockholm University) at 10 micromolar (µM) concentrations were used as a treatment modality. They were diluted 1,000× from the stock in the dimethyl sulfoxide (DMSO) solution.
Ethical clearance
The use of zebrafish embryos that are younger than 5 days is exempted from ethical approvals as they are not considered research animals according to FELASA and Swedish rules. Notwithstanding, the investigator is certified to work with both adult and embryonic zebrafish, and the concerned lab (Linkoping University) has ethical approval for research studies related to zebrafish, including tumor implantation studies (N89/15).
Statistical analysis
Statistical differences between the groups were determined by Student’s t-test using GraphPad Prism Software (GraphPad Software, San Diego, CA, USA) and P<0.05 was considered statistically significant. Tumor areas after injection of PANC-1 cell line in the PVS of zebrafish embryos (ZE) without (Control group) and with Sirt6 (+ Sirt6 inhibitor/analog group) were depicted as Whisker’s plots using GraphPad Prism Software (GraphPad Software, San Diego, CA, USA) and P<0.05 was considered statistically significant.
Results
We studied the effect of HDAC6 inhibitors on pancreatic cancer tumor regression and its ability to inhibit metastasis employing zebrafish embryos. The human cell line PANC-1 was injected into the PVS of zebrafish embryos. Since the process required expertise, we repeated experiments until successful and reproducible results were achieved. In addition, we selected only those embryos where the cells were implanted in the PVS only and not in the yolk sack. Around 120–150 embryos were injected in each experiment.
Three days of after implantation, the area of the tumor increased, a representative fluorescent micrograph of day 0 and day 3 is shown in Figure 1.
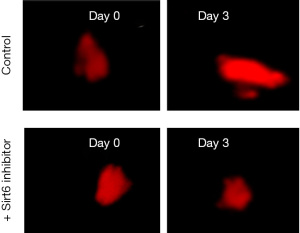
Sirt6 inhibitors at 10 micromolar (µM) were used. After treatment with Sirt6 inhibitors (+ Sirt6 inhibitor group), there was a reduction in the growth of the tumor from day 0 to day 3 as shown in Figure 1. We then measured the tumor areas on day 3 of the Control and + Sirt6 inhibitor group and observed a significant (P=0.001) reduction in the tumor size of the later (Figure 2 and Table 1).
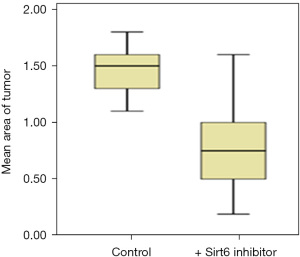
Table 1
Cell line | Tumor area (µm2) day 3 after implantation | P value* | |
---|---|---|---|
Control group | + Sirt6 inhibitor group | ||
PANC-1 | 1.5 (1.3–1.6) | 0.75 (0.5–1.0) | 0.001 |
Data are expressed as median (interquartile range). *, Student’s t-test. PANC-1, pancreatic adenocarcinoma.
Pancreatic duct adenocarcinoma is characterized by early metastasis. We, therefore, studied the level of metastasis in PANC-1 cell line after fertilization in the Control and the group treated with Sirt6 inhibitor. Red fluorescent labeled PANC-1 cells were thus injected into the PVS of zebrafish embryos at 48 hours post-fertilization as a Control group. The injected cells could successfully form a solid tumor at the site of the injection, and it also showed a level of metastasis with a varying number of cells migrated from the PVS to the tail region (Figures 3,4). Simultaneously, in another set of experiments PANC-1 cells were microinjected into zebrafish embryos post-fertilization and treated with Sirt6 inhibitor. About 30 embryos were used per experiment. Here we observed a lower level of metastasis as compared to the Control group (Figure 5 and Table 2).
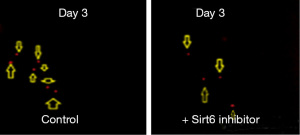
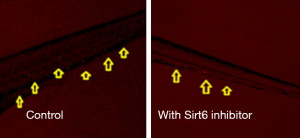
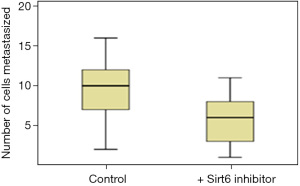
Table 2
Cell line | No. of cells metastasized | P value* | |
---|---|---|---|
Control group | Group with Sirt6 inhibitor | ||
PANC-1 | 10 [6–13] | 6 [3–8] | 0.001 |
Data are expressed as median [interquartile range]. *, Student’s t-test. PANC-1, pancreatic adenocarcinoma.
We further tested the effect of the analog of Sirt6 on the tumor. Subsequent to the implantation of the tumor, 10 µmolar (µM) of Sirt6 analog was injected and 3 days later area of the tumor was compared with the area of tumor seen on day 0 (Figure 6). A significant regression of tumor area (P<0.0001) was observed (Figure 7), and the experiment was repeated twice (Table 3).
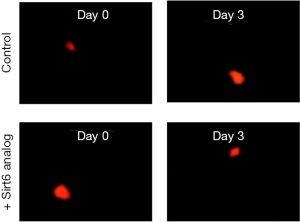
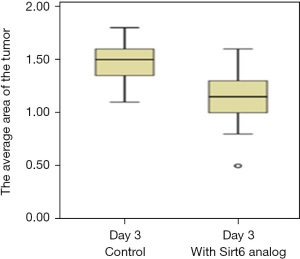
Table 3
Cell line | Tumor area (µm2), day 3 after implantation | P value* | |
---|---|---|---|
Control group | + Sirt6 analog | ||
PANC-1 | 1.5 (1.3–1.6) | 1.15 (1–1.3) | 0.001 |
Data are expressed as median (interquartile range). *, Student’s t-test. PANC-1, pancreatic adenocarcinoma.
The anti-metastatic activity of the Sirt6 analog on the PANC-1 cell line was also assessed. A group of zebrafish embryos received Sirt6 analog following the procedure as described for studying the effect of Sirt6 inhibitor. A representative fluorescent micrograph showing metastasized on day 3 is shown in Figure 8. The number of cells that migrated from the PVS to the tail was few in the group treated with the Sirt6 analog as compared to the Control (Figure 9). The group treated with Sirt6 analog showed a significant (P=0.001) decrease in the number of cells undergoing metastasis as compared to the Control group (Figure 10 and Table 4).

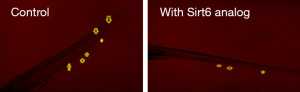
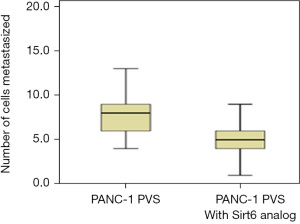
Table 4
Cell line | No. of cells metastasized | P value* | |
---|---|---|---|
Control group | Group with Sirt6 analog | ||
PANC-1 | 8 [6–9] | 5 [4–6] | 0.001 |
Data are expressed as median [interquartile range]. *, Student’s t-test. PANC-1, pancreatic adenocarcinoma.
Discussion
Histone acetylation and deacetylation are important epigenetic mechanisms, and HDAC6 is a member of the HDAC family. Besides deacetylating histones, it targets non-histone substrates and regulates cell proliferation, metastasis, cancer cell migration and invasion, and mitosis in tumors (26-28). In addition, it upregulates several important factors in the immune system, which are the primary targets of cancer immunotherapy (29,30). These features make HDAC6 a promising target in cancer drug development. In this study, we aimed to decipher the role of histone acetaldehyde inhibitor in pancreatic cancer tumor regression and its ability to inhibit metastasis and chose the zebrafish model.
The use of zebrafish as an animal model for studying tumor biology has increased recently (31,32). The utilization of transparent zebrafish embryos, with fluorescent endothelial and or immune cells, makes possible the visualization of cell-to-cell interaction, within host cells themselves and between zebrafish stroma and the human cancer cells implanted (33,34). Studies have shown that there is high conservation of tumor-suppressor genes and oncogenes between zebrafish and human, making data elaborated in zebrafish essential for understanding human diseases (35).
In this study, human tumor cells were engrafted into the PVS of the zebrafish embryos for the inducement of primary tumor growth and metastasis. Induction of angiogenesis through tumor, the formation of metastasis, inhibition of these processes, and other comparative chemical frameworks have been studied extensively using the zebrafish metastasis model (36). The zebrafish metastasis model offers the opportunity to explain critical kinetics and main frameworks that are involved in the regulation of tumor-microenvironment interactions and support tumor spreading (33,37).
It has been reported that the regulation of cancer extravasation is through fibroblasts and mesenchymal cells present in the tumor microenvironment (38). The study by Liu et al. [2017] revealed that cancer-related fibroblasts increased cancer cell metastasis at the very early stage during primary tumor advancement (37). Cancer-related fibroblasts can hijack cancer cells for spreading from the primary site even at a stage where a primary tumor is of a microscopic size with only a few hundred cells (37). In this study, three days after implantation of cells, the average areas of tumors formed increased significantly from day 0 to day 3 in the Control group. We also observed cancer metastasis which is typified by abnormal cell migration to the tail as reported earlier (39).
We further examined the involvement of Sirt6 in cancer biology and therefore used Sirt6 inhibitor to see the anticancer effects of Sirt6 inhibition on the PANC-1 cell lines injected in the PVS of the zebrafish embryos. The anticancer operations of histone deacetylase inhibitors (HDACi) have been reported by various studies and found that they arrest the cell cycle and cause inhibition of angiogenesis (13). Our study showed that there was a significant difference between the Control and the treatment groups. Significant decreases in the average area of tumor were observed in the treatment groups, PANC-1 PVS with Sirt6 inhibitors, when compared with the Control groups, 3 days of treatment, indicating that Sirt6 elicited anticancer effects.
Three days after implantation of cells, the tumor area regressed significantly when compared with the Control, indicating that Sirt6 inhibitor elicited anticancer effects. Besides regression of tumor area, it also caused significant decreases in the number of cells undergoing metastasis. One of the probable mechanisms involved is that the inhibitor regulates the acetylation state of histone; and interferes with the balance of histone acetylation and deacetylation in tumor cells, thus inhibiting tumor cell angiogenesis invasion, and metastasis (40,41). In agreement with our observation, a previous study has explored the anticancer activities of Sirt6 inhibitors has reported arresting the cell cycle and causing inhibition of angiogenesis (13). Our work is in agreement with the findings reported previously. A study that used tubacin, a selective HDAC6i, to examine the effect of pancreatic tumor growth in zebrafish models reported that HDAC6i was linked to the deacetylation of α-tubulin in a zebrafish model (42). Another study using a selective inhibitor of class I and II HDACs with ML-60218 revealed that this combination elicited synergistic inhibition of proliferation of tumors by initiating cell cycle arrest and upregulation of CDK-inhibitors (42).
It was also found that there was a significant decrease in the number of metastasized cells in the treatment group when compared with the Control group after 3 days of treatment. Our findings support that Sirt6 inhibitors could be used as anticancer drugs targeting both proliferation and invasion and metastasis at the same time.
Further, isoform-specific inhibitors of its enzymatic have also been synthesized, and some of these inhibitors are currently in clinical trials (43,44). We also demonstrated that Sirt6 analog could restrict the growth of tumor size and reduce the number of cells undergoing metastasis.
There is a staggering unmet clinical need to create new drugs for the treatment of pancreatic cancer. To date, the focus on the medical treatment of this disease is sparse, and fruitful clinical outcome of the research is yet to be shown. The data on the role of HDAC6 as a target for the treatment of pancreatic cancer in zebrafish models is very limited. Towards this end, our work provides the basis for the use of HDAC6 inhibitors in clinical practices.
Limitations of the study
The study limitations include the duration of the implantation and injecting inhibitor or its analog of three days. An extended study duration can be performed to observe the long-term effects of the implanted cells concerning the reduction in tumor size and the effects of the inhibitor as a potential therapeutic agent. Also, any translational studies in cancer require further confirmation of the results by performing more assays like Western blotting, real-time polymerase chain reaction (RT-PCR), immunohistochemistry (IHC) analysis, and flow cytometry which is a limitation in this study.
Conclusions
The present study could confirm the potency of HDAC6 inhibitor and its analog to regress tumor size and reduce the number of cells undergoing metastasis. The focus on the tumor microenvironment and extravasation is a requirement to research the migration and proliferation of these cells and understand their signaling pathways. Further, isoform-specific inhibitors of the enzyme have also been synthesized, and some of these inhibitors are currently in clinical trials. It paves a new direction for using these inhibitors as therapeutic agents and calls for more translational-based research with a larger scale and optimum dosage range to be studied to be utilized on a commercial scale.
Acknowledgments
My sincere thanks to my supervisor Dr. Lasse Jensen (Senior Association Professor at Linkoping University) for his expertise, ideas, feedback, and encouragement. Also, deep regards to my mentor, Dr. Jonas Wetterö (Associate Professor at Linkoping University) for all guidance throughout the work.
Funding: This work was financially supported by
Footnote
Reporting Checklist: The author has completed the STROBE reporting checklist. Available at https://pcm.amegroups.com/article/view/10.21037/pcm-23-8/rc
Data Sharing Statement: Available at https://pcm.amegroups.com/article/view/10.21037/pcm-23-8/dss
Peer Review File: Available at https://pcm.amegroups.com/article/view/10.21037/pcm-23-8/prf
Conflicts of Interest: The author has completed the ICMJE uniform disclosure form (available at https://pcm.amegroups.com/article/view/10.21037/pcm-23-8/coif). The author has no conflicts of interest to declare.
Ethical Statement: The author is accountable for all aspects of the work in ensuring that questions related to the accuracy or integrity of any part of the work are appropriately investigated and resolved. The use of zebrafish embryos that are younger than five days is exempted from ethical approvals as they are not considered research animals according to FELASA and Swedish rules. Notwithstanding, the investigator is certified to work with both adult and embryonic zebrafish, and the concerned lab (Linkoping University) has ethical approval for research studies related to zebrafish, including tumor implantation studies (N89/15).
Open Access Statement: This is an Open Access article distributed in accordance with the Creative Commons Attribution-NonCommercial-NoDerivs 4.0 International License (CC BY-NC-ND 4.0), which permits the non-commercial replication and distribution of the article with the strict proviso that no changes or edits are made and the original work is properly cited (including links to both the formal publication through the relevant DOI and the license). See: https://creativecommons.org/licenses/by-nc-nd/4.0/.
References
- Ferlay J, Colombet M, Soerjomataram I, et al. Estimating the global cancer incidence and mortality in 2018: GLOBOCAN sources and methods. Int J Cancer 2019;144:1941-53. [Crossref] [PubMed]
- de Martel C, Georges D, Bray F, et al. Global burden of cancer attributable to infections in 2018: a worldwide incidence analysis. Lancet Glob Health 2020;8:e180-90. [Crossref] [PubMed]
- Ferlay J, Colombet M, Soerjomataram I, et al. Cancer incidence and mortality patterns in Europe: Estimates for 40 countries and 25 major cancers in 2018. Eur J Cancer 2018;103:356-87. [Crossref] [PubMed]
- Rawla P, Sunkara T, Gaduputi V. Epidemiology of Pancreatic Cancer: Global Trends, Etiology and Risk Factors. World J Oncol 2019;10:10-27. [Crossref] [PubMed]
- Yee NS, Pack M. Zebrafish as a model for pancreatic cancer research. Methods Mol Med 2005;103:273-98. [PubMed]
- Javadrashid D, Baghbanzadeh A, Derakhshani A, et al. Pancreatic Cancer Signaling Pathways, Genetic Alterations, and Tumor Microenvironment: The Barriers Affecting the Method of Treatment. Biomedicines 2021;9:373. [Crossref] [PubMed]
- Hanahan D. Hallmarks of Cancer: New Dimensions. Cancer Discov 2022;12:31-46. [Crossref] [PubMed]
- Schnekenburger M, Florean C, Dicato M, et al. Epigenetic alterations as a universal feature of cancer hallmarks and a promising target for personalized treatments. Curr Top Med Chem 2016;16:745-76. [Crossref] [PubMed]
- Zhang J, Zhong Q. Histone deacetylase inhibitors and cell death. Cell Mol Life Sci 2014;71:3885-901. [Crossref] [PubMed]
- Ouaïssi M, Giger U, Sielezneff I, et al. Rationale for possible targeting of histone deacetylase signaling in cancer diseases with a special reference to pancreatic cancer. J Biomed Biotechnol 2011;2011:315939. [PubMed]
- Ustinova K, Novakova Z, Saito M, et al. The disordered N-terminus of HDAC6 is a microtubule-binding domain critical for efficient tubulin deacetylation. J Biol Chem 2020;295:2614-28. [Crossref] [PubMed]
- Li T, Zhang C, Hassan S, et al. Histone deacetylase 6 in cancer. J Hematol Oncol 2018;11:111. [Crossref] [PubMed]
- Dallavalle S, Pisano C, Zunino F. Development and therapeutic impact of HDAC6-selective inhibitors. Biochem Pharmacol 2012;84:756-65. [Crossref] [PubMed]
- Rivieccio MA, Brochier C, Willis DE, et al. HDAC6 is a target for protection and regeneration following injury in the nervous system. Proc Natl Acad Sci U S A 2009;106:19599-604. [Crossref] [PubMed]
- Eckschlager T, Plch J, Stiborova M, et al. Histone Deacetylase Inhibitors as Anticancer Drugs. Int J Mol Sci 2017;18:1414. [Crossref] [PubMed]
- Streisinger G, Walker C, Dower N, et al. Production of clones of homozygous diploid zebra fish (Brachydanio rerio). Nature 1981;291:293-6. [Crossref] [PubMed]
- Bardeesy N, Sharpless NE, DePinho RA, et al. The genetics of pancreatic adenocarcinoma: a roadmap for a mouse model. Semin Cancer Biol 2001;11:201-18. [Crossref] [PubMed]
- Penberthy WT, Shafizadeh E, Lin S. The zebrafish as a model for human disease. Front Biosci 2002;7:d1439-53. [Crossref] [PubMed]
- Teame T, Zhang Z, Ran C, et al. The use of zebrafish (Danio rerio) as biomedical models. Anim Front 2019;9:68-77. [Crossref] [PubMed]
- Warga RM, Nüsslein-Volhard C. Origin and development of the zebrafish endoderm. Development 1999;126:827-38. [Crossref] [PubMed]
- Ward AB, Warga RM, Prince VE. Origin of the zebrafish endocrine and exocrine pancreas. Dev Dyn 2007;236:1558-69. [Crossref] [PubMed]
- Kroon E, Martinson LA, Kadoya K, et al. Pancreatic endoderm derived from human embryonic stem cells generates glucose-responsive insulin-secreting cells in vivo. Nat Biotechnol 2008;26:443-52. [Crossref] [PubMed]
- Matsuda H. Zebrafish as a model for studying functional pancreatic β cells development and regeneration. Dev Growth Differ 2018;60:393-9. [Crossref] [PubMed]
- Field HA, Dong PD, Beis D, et al. Formation of the digestive system in zebrafish. II. Pancreas morphogenesis. Dev Biol 2003;261:197-208. [Crossref] [PubMed]
- Chung WS, Andersson O, Row R, et al. Suppression of Alk8-mediated Bmp signaling cell-autonomously induces pancreatic beta-cells in zebrafish. Proc Natl Acad Sci U S A 2010;107:1142-7. [Crossref] [PubMed]
- Lee YS, Lim KH, Guo X, et al. The cytoplasmic deacetylase HDAC6 is required for efficient oncogenic tumorigenesis. Cancer Res 2008;68:7561-9. [Crossref] [PubMed]
- Wickström SA, Masoumi KC, Khochbin S, et al. CYLD negatively regulates cell-cycle progression by inactivating HDAC6 and increasing the levels of acetylated tubulin. EMBO J 2010;29:131-44. [Crossref] [PubMed]
- Lafarga V, Aymerich I, Tapia O, et al. A novel GRK2/HDAC6 interaction modulates cell spreading and motility. EMBO J 2012;31:856-69. [Crossref] [PubMed]
- Woan KV, Lienlaf M, Perez-Villaroel P, et al. Targeting histone deacetylase 6 mediates a dual anti-melanoma effect: Enhanced antitumor immunity and impaired cell proliferation. Mol Oncol 2015;9:1447-57. [Crossref] [PubMed]
- M L. Essential role of HDAC6 in the regulation of PD-L1 in melanoma. Mol Oncol 2016;10:735-50. [Crossref] [PubMed]
- Rouhi P, Jensen LD, Cao Z, et al. Hypoxia-induced metastasis model in embryonic zebrafish. Nat Protoc 2010;5:1911-8. [Crossref] [PubMed]
- Hason M, Bartůněk P. Zebrafish Models of Cancer-New Insights on Modeling Human Cancer in a Non-Mammalian Vertebrate. Genes (Basel) 2019;10:935. [Crossref] [PubMed]
- Tulotta C, Groenewoud A, Snaar-Jagalska BE, et al. Animal Models of Breast Cancer Bone Metastasis. Methods Mol Biol 2019;1914:309-30. [Crossref] [PubMed]
- Ellett F, Pase L, Hayman JW, et al. mpeg1 promoter transgenes direct macrophage-lineage expression in zebrafish. Blood 2011;117:e49-56. [Crossref] [PubMed]
- Ung CY, Lam SH, Gong Z. Comparative transcriptome analyses revealed conserved biological and transcription factor target modules between the zebrafish and human tumors. Zebrafish 2009;6:425-31. [Crossref] [PubMed]
- Tulotta C, He S, van der Ent W, et al. Imaging Cancer Angiogenesis and Metastasis in a Zebrafish Embryo Model. Adv Exp Med Biol 2016;916:239-63. [Crossref] [PubMed]
- Liu C, Zhang Y, Lim S, et al. A Zebrafish Model Discovers a Novel Mechanism of Stromal Fibroblast-Mediated Cancer Metastasis. Clin Cancer Res 2017;23:4769-79. [Crossref] [PubMed]
- Correa D, Somoza RA, Lin P, et al. Mesenchymal stem cells regulate melanoma cancer cells extravasation to bone and liver at their perivascular niche. Int J Cancer 2016;138:417-27. [Crossref] [PubMed]
- Suhail Y, Cain MP, Vanaja K, et al. Systems Biology of Cancer Metastasis. Cell Syst 2019;9:109-27. [Crossref] [PubMed]
- Wei Z, Shan Y, Tao L, et al. Diallyl trisulfides, a natural histone deacetylase inhibitor, attenuate HIF-1α synthesis, and decreases breast cancer metastasis. Mol Carcinog 2017;56:2317-31. [Crossref] [PubMed]
- Abe F, Kitadate A, Ikeda S, et al. Histone deacetylase inhibitors inhibit metastasis by restoring a tumor suppressive microRNA-150 in advanced cutaneous T-cell lymphoma. Oncotarget 2017;8:7572-85. [Crossref] [PubMed]
- Hideshima T, Bradner JE, Wong J, et al. Small-molecule inhibition of proteasome and aggresome function induces synergistic antitumor activity in multiple myeloma. Proc Natl Acad Sci U S A 2005;102:8567-72. [Crossref] [PubMed]
- Vogl DT, Raje N, Jagannath S, et al. Ricolinostat, the First Selective Histone Deacetylase 6 Inhibitor, in Combination with Bortezomib and Dexamethasone for Relapsed or Refractory Multiple Myeloma. Clin Cancer Res 2017;23:3307-15. [Crossref] [PubMed]
- Tu Y, Hershman DL, Bhalla K, et al. A phase I-II study of the histone deacetylase inhibitor vorinostat plus sequential weekly paclitaxel and doxorubicin-cyclophosphamide in locally advanced breast cancer. Breast Cancer Res Treat 2014;146:145-52. [Crossref] [PubMed]
Cite this article as: Helmi M. Evaluation of the anti-tumor activity of histone deacetylase inhibitors (HDAC6) on human pancreatic adenocarcinoma (PANC-1) cell line using a zebrafish xenograft model. Precis Cancer Med 2024;7:5.