User:Marshallsumter/Radiation astronomy/Craters

A crater may be any large, roughly circular, depression or hole in or beneath the rocky surface of a rocky object.
Crater astronomy applies the techniques of astronomy to the apparent craters observed on rocky objects in an effort to understand what they are, when they occurred, and their importance to rocky objects.
Minerals
[edit | edit source]


Alpha-quartz (space group P3121, no. 152, or P3221, no. 154) under a high pressure of 2-3 gigapascals and a moderately high temperature of 700°C changes space group to monoclinic C2/c, no. 15, and becomes the mineral coesite. It is "found in extreme conditions such as the impact craters of meteorites.
Shocked quartz is associated with two high pressure polymorphs of silicon dioxide: coesite and stishovite. These polymorphs have a crystal structure different from standard quartz. Again, this structure can only be formed by intense pressure, but moderate temperatures. High temperatures would anneal the quartz back to its standard form. Stishovite may be formed by an instantaneous over pressure such as by an impact or nuclear explosion type event.
So far several of the polymorphs of α-quartz formed at high temperature and pressure occur with rock types away from meteorite impact craters.
At lower left is a thin section through a sand-sized quartz grain "from the USGS-NASA Langley core showing two well-developed, intersecting sets of shock lamellae produced by the late Eocene Chesapeake Bay bolide impact. This shocked quartz grain is from the upper part of the crater-fill deposits at a depth of 820.6 ft in the core. The corehole is located at the NASA Langley Research Center, Hampton, VA, near the southwestern margin of the Chesapeake Bay impact crater."[1] "Very high pressures produced by strong shock waves cause dislocations in the crystal structure of quartz grains along preferred orientations. These dislocations appear as sets of parallel lamellae in the quartz when viewed with a petrographic microscope. Bolide impacts are the only natural process known to produce shock lamellae in quartz grains."[1]
Lower right shows another thin section in plane polarized light of a shocked quartz grain with two sets of decorated planar deformation features (PDFs) surrounded by a cryptocrystalline matrix from the Suvasvesi South impact structure, Finland.
In a specimen of shocked quartz, stishovite can be separated from quartz by applying hydrogen fluoride (HF); unlike quartz, stishovite will not react.[2]
Minute amounts of stishovite has been found within diamonds.[3]
The major evidence for a volcanic origin for tektites "includes: close analogy between shaped tektites and small volcanic bombs, and between layered tektites and lava or tuff-lava flows or huge bombs; analogy between flanged tektites and volcanic bombs ablated by gasjets: long-time, multistage formation of some tektites that corresponds to wide variations in their radiometric ages; well-ordered long compositional trends (series) typical of magmatic differentiation; different compositional tektite families (subseries) comparable to different stages (phases) of the volcanic process."[4]
"As with the North American microtektite-bearing cores, all the Australasian microtektite-bearing cores containing coesite and shocked quartz also contained volcanic ash, which complicated the search."[5]
Theoretical crater astronomy
[edit | edit source]A "cataclysm [may] have affected the entire inner Solar System ... [when] numerous main-belt asteroids ... were driven onto high-velocity and highly eccentric orbits by the effects of the late migration of the giant planets."[6]
The sequence of figures depict a likely model for caldera collapse.
A collapse is triggered by the emptying of the magma chamber beneath the volcano, usually as the result of a large volcanic eruption. If enough magma is ejected, the emptied chamber is unable to support the weight of the volcanic edifice above it. A roughly circular fracture, the ring fault, develops around the edge of the chamber. Ring fractures serve as feeders for fault intrusions which are also known as ring dykes. Secondary volcanic vents may form above the ring fracture. As the magma chamber empties, the center of the volcano within the ring fracture begins to collapse. The collapse may occur as the result of a single cataclysmic eruption, or it may occur in stages as the result of a series of eruptions. The total area that collapses may be hundreds or thousands of square kilometers.
Catenae are thought to have been formed by the impact of a body that was broken up by tidal forces into a string of smaller objects following roughly the same orbit. An example of such a tidally disrupted body that was observed prior to its impact on Jupiter is Comet Shoemaker-Levy 9.
Catenae on Mars, represent chains of collapse pits associated with grabens (see, for example, the Tithoniae Catenae near Tithonium Chasma).
Crater chains seen on the Moon often radiate from larger craters, and in such cases are thought to be either caused by secondary impacts of the larger crater's ejecta or by volcanic venting activity along a rift.[7]
Entities
[edit | edit source]
Def.
- the study of rock layers and the layering process of stratification or
- the layering of deposits, with newer remains overlaying older ones, forming a chronology of the site
is called stratigraphy.
Def. the process leading to the formation or deposition of layers, especially of sedimentary rocks is called stratification.
Def.
- one of several parallel horizontal layers of material arranged one on top of another,
- a layer of sedimentary rock having approximately the same composition throughout, or
- any of the regions of the atmosphere, such as the stratosphere, that occur as layers
is called a stratum, plural strata.
Natural bombs may produce impact craters and deform rock strata.
"There is no correlation between n (the exponent for the change in particle diameter) and any of the other entities, which shows that n-values cannot be used as an index of maturation of a soil."[8]
where is change in particle diameter, is the constant of surface area rate of change, is the measured particle diameter in the soil, is the initial particle diameter for all samples, and is the exponent.
Sources
[edit | edit source]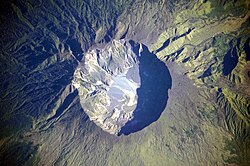

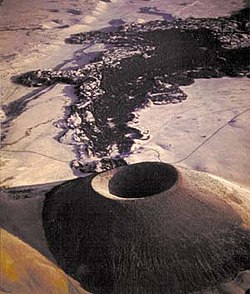
The first image at right is a "detailed astronaut photograph [that] depicts the summit caldera of the volcano. The huge caldera—6 kilometers (3.7 miles) in diameter and 1,100 meters (3,609 feet) deep—formed when Tambora’s estimated 4,000-meter- (13,123-foot) high peak was removed, and the magma chamber below emptied during the April 10 eruption. Today the crater floor is occupied by an ephemeral freshwater lake, recent sedimentary deposits, and minor lava flows and domes from the nineteenth and twentieth centuries. Layered tephra deposits are visible along the northwestern crater rim. Active fumaroles, or steam vents, still exist in the caldera."[9]
"On April 10, 1815, the Tambora Volcano produced the largest eruption in recorded history. An estimated 150 cubic kilometers (36 cubic miles) of tephra—exploded rock and ash—resulted, with ash from the eruption recognized at least 1,300 kilometers (808 miles) away to the northwest. While the April 10 eruption was catastrophic, historical records and geological analysis of eruption deposits indicate that the volcano had been active between 1812 and 1815. Enough ash was put into the atmosphere from the April 10 eruption to reduce incident sunlight on the Earth’s surface, causing global cooling, which resulted in the 1816 “year without a summer.”"[9]
At right is the crater in Santa Ana Volcano is photographed from a United States Air Force C-130 Hercules flying above El Salvador.
A volcanic crater is a circular depression in the ground caused by volcanic activity.[10] It is typically a basin, circular in form within which occurs a vent (or vents) from which magma erupts as gases, lava, and ejecta. A crater can be of large dimensions, and sometimes of great depth. During certain types of climactic eruptions, the volcano's magma chamber may empty enough for an area above it to subside, forming what may appear to be a crater but is actually known as a caldera.
In the majority of typical volcanoes, the crater is situated atop the mountain formed from the erupted volcanic deposits such as lava flows and tephra. Volcanoes that terminate in such a summit crater are usually of a conical form. Other volcanic craters may be found on the flanks of volcanoes, and these are commonly referred to as flank craters. Some volcanic craters may fill either fully or partially with rain and/or melted snow, forming a crater lake.
The second image at right shows a solidified lava lake that composes the floor of the Karthala volcano crater.
Phreatic eruptions typically include steam and rock fragments; the inclusion of lava is unusual. The temperature of the fragments can range from cold to incandescent. If molten material is included, the term phreato-magmatic may be used. These eruptions occasionally create broad, low-relief craters called maars.
S P Crater is a cinder cone volcano in the San Francisco volcanic field, 25 miles (40 km) north of Flagstaff, Arizona.[11] It is surrounded by several other cinder cones which are older and more eroded. It is a striking feature on the local landscape, with a well-defined lava flow that extends for 7 kilometers (4.3 mi) to the north.[12]
S P Crater is a 820 foot high cinder cone of basaltic andesite. The cone is capped by an agglutinate rim that helps to protect its structure. A lava flow extends to the north of the cone for ~7 km and originated from the same vent.[13] Some workers consider the lava flow to have slightly predated the cinder cone because of geochemical data that suggests the flow is more silica rich than the cinders and based on the observation that the cone overlaps the lava flow and shows no sign of deformation.[13] However, there is some debate about the relationship between the cone and flow as it is not uncommon to form cinder cones during the early phase of an eruption as a magma degasses, and then to have lava push through the side of a cone during a late phase of eruption.
K/Ar dates on the lava are ~ 70 ka,[14] but are considered unreliable because of excess Ar[15] and the un-weathered young appearance of the cone.
"Independently of other criteria the distribution of the KIT boundary ejecta predicts that the Chicxulub crater is the K/T source crater."[16]
"In agreement with many authors (Pal et al., 1982; Klein and Middleton, 1984; Blum et al., 1992), we therefore exclude meteoritic and lunar material as sources for the 10Be in the Australasian tektites, and, by a short extension, for virtually all the other atoms in the tektites."[17]
Objects
[edit | edit source]
Def. a hemispherical pit, a basinlike opening or mouth about which a cone is often built up, any large roughly circular depression or hole is called a crater.
Secondary craters are impact craters formed by the ejecta that was thrown out of a larger crater. They sometimes form radial crater chains.
"The present paper deals with the problem of estimating the flux of objects large enough to produce impact craters on earth."[18]
"Phoebe is a densely cratered object. Cumulative numbers of craters, between 100 m and 100 km diameter, per unit area, define a steep-sloped curve (Fig. 5). Crater densities approach those seen on other heavily cratered objects (15, 16)."[19]
Strong forces
[edit | edit source]


A caldera is a cauldron-like volcanic feature usually formed by the collapse of land following a volcanic eruption. They are sometimes confused with volcanic craters. The word comes from Spanish caldera, and this from Latin CALDARIA, meaning "cooking pot". In some texts the English term cauldron is also used.
About 75,000 years ago, this Indonesian volcano released about 2,800 km3 DRE of ejecta, the largest known eruption within the Quaternary Period (last 1.8 million years) and the largest known explosive eruption within the last 25 million years. In the late 1990s, anthropologist Stanley Ambrose[20] proposed that a volcanic winter induced by this eruption reduced the human population to about 2,000 - 20,000 individuals, resulting in a population bottleneck (see Toba catastrophe theory). More recently several geneticists, including Lynn Jorde and Henry Harpending have proposed that the human race was reduced to approximately five to ten thousand people.[21] Whichever figure is right, the fact remains that the human race seemingly came close to extinction about 75,000 years ago.
Electromagnetics
[edit | edit source]
The image at page top right shows a chain of 13 craters (Enki Catena) on Ganymede measuring 161.3 km in length. The Enki craters formed across the sharp boundary between areas of bright terrain and dark terrain, delimited by a thin trough running diagonally across the center of this image. The ejecta deposit surrounding the craters appears very bright on the bright terrain. Even though all the craters formed nearly simultaneously, it is difficult to discern any ejecta deposit on the dark terrain.
A crater chain is a line of craters along the surface of an astronomical body. The descriptor term for crater chains is catena (plural catenae), as specified by the International Astronomical Union's rules on planetary nomenclature.[22]
"The Davy crater chain (arrow) is one of the most spectacular chains of craters on the Moon. It extends for about 50 km across the floor of the large, very old crater Davy Y (Y) and onto its eastern rim. The chain may be related in origin to the pair of irregular craters Davy G (G) and Davy GA (GA), 75 km from the furthest end of the chain. Two origins have been proposed. Some lunar geologists believe it is a chain of secondary impact craters, and others believe it is a line of volcanic craters. The simple geometry of the Davy chain, the symmetry and uniform spacing of its individual craters, and its alinement with Davy G strongly support, in this writer's opinion, a volcanic origin. Also arguing against a secondary impact origin is the fact that the Davy chain is a lone feature. There are no other similar chains with this trend in the area. As was shown earlier in this chapter (figs. 124 and 125), secondary crater chains tend to occur in large numbers within the belt of secondary craters surrounding a large primary crater."[23]
“Electrical currents flow in [plasmas], punching into long filaments and then braiding themselves into ropelike structures. These long, twisted filaments are visible in solar prominences, galactic jets, and comet tails. They were detected as “stringy things” in the forty-million-kilometer-long tail of Venus last year.”[24]
“[M]ost features now being photographed on planets, satellites, and asteroids are scars of plasma discharges: interplanetary lightning.”[24]
“[A] high-voltage pointed rod [moving] just above the surface of a powder-covered insulator placed on a grounded metal plate ... [sparks to form] ... [a] long, narrow main channel of fairly uniform width [with] a narrower, more sinuous channel engraved along its center. Tributary channels run parallel to the main channel for a distance, then they rejoin it almost perpendicularly.”[24]
“Neither water nor lava produces channels puntuated by circular pits, but this is a common feature of discharge channels”[24].
“There is generally no sign of rubble from collapsed roofs, as would be expected if the conventional “lava tube” explanation for rilles on the Moon [is] correct.”[24]
“[A]n electrical arc always strikes perpendicular to a surface [creating perfectly circular craters].[24] “[T]erracing along the sides, sometimes [corkscrew] down to the [crater] floor, following the rotary motion of [an electric] arc”[24] “Central peaks tend to be symmetrical and steep-sided, similar to the central “nipple” left by plasma machining as the rotary corkscrewing motion of the arc cuts out the material around it.”“[T]erracing along the sides, sometimes [corkscrew] down to the [crater] floor, following the rotary motion of [an electric] arc”[24].
“In a number of craters on the Moon, the central peak connects to the surrounding terrain with an “isthmus,” just as in a plasma-machined crater when the arc is quenched before completing a full rotation. A telling characteristic of electrical origin ... is a crater centered on the rim of another crater. This is a common sight on the Moon and some planets. It is an expected effect of the arc jumping to or striking the highest elevations.”[24]
“Impact and explosion craters ... tend to have a bowl shape”[24] “[T]he irregular mass [is created by] the so-called rebound peak in a lab-produced impact or explosion crater.”[24]
"Several years after Dietz's paper, Ford used a spark-machining apparatus and demonstrated similarities between laboratory craters and lunar craters [2]."[25]
A superbolt, more powerful than an ordinary lightning bolt, struck a cornfield near Leland, Illinois, leaving a crater one foot deep, and breaking windows in homes almost a mile away.[26]
Continua
[edit | edit source]"Croft [3] called moat craters anomalous pit craters and also suggested a continuum between moat craters, craters, and palimpsests, even though morphometrically they appeared to be distinct."[27]
Emissions
[edit | edit source]



A resurgent dome forms the island of Samosir within the caldera of Lake Toba. It is 100 km/62 mi long and 30 km/19 mi wide, a caldera of the world's largest class.
When the material above the explosion is solid rock, then a mound may be formed by broken rock that has a greater volume. This type of mound has been called "retarc", "crater" spelled backwards.[28]
Hár is a crater on Jupiter's moon Callisto. Its name is one of the many names of Odin, the supreme god in Norse mythology. This is an example of a central dome impact crater.[29]
"The name Panum Crater refers to a crater surrounded by [an] ejecta ring, with a dome in the middle. At Panum Crater the dome didn't completely fill the crater or overrun the ring (as often happens) providing an opportunity to explore all three structures."[30]
"At Panum, a pyroclastic eruption (new magma explosively fragmented into the air) followed the phreatic (steam) eruption. During a pyroclastic eruption, the gas within the magma continues to expand and escape as the magma is thrown into the air and cools. The resulting deposits included ash (particles <2mm in size) and pumice. The pumice is frothy preserving the frozen gas bubbles."[30]
"The ejecta ring is made up of small bits of pumice, ash, obsidian fragments, and well-rounded granitic pebbles (which were part of the surrounding rock and not formed during the eruption) that were ejected during the final explosive stage of the eruption."[30]
"The central lava dome was erupted from degassed material and is made up of pumice and obsidian of the same composition. The difference between the two has to do with gas escaping as the magma cooled. The magma that created the dome had dissolved gas in it, like a bottle of seltzer water. As the magma rose towards the surface where there was less pressure on it than at depth, the gas expanded producing the holes (bubbles) you see in the pumice. The magma that remained pressurized while it cooled quickly or that had already lost its gas, formed the obsidian."[30]
"Flow banding containing both obsidian and pumice is common at Panum Crater. Another common texture, called breadcrust, can also be seen in the dome. Breadcrust textures form when the inside of a cooling rock is still hot with gas escaping from it while the outside surface has already cooled. As the gas expands from the inside, the outside surface cracks to allow the gas to escape."[30]
Absorptions
[edit | edit source]


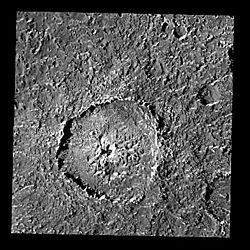



The image at right shows the crater created by the Sedan shallow underground nuclear test explosion.
At left is a stylised cross-section of a crater formed by a below-ground explosion.
A crater is formed by an explosive event through the displacement and ejection of material from the ground. It is typically bowl-shaped. High pressure gas and pressure waves are responsible for the creation of the crater by three processes
- plastic deformation of the ground
- projection of material (ejecta) from the ground by the expansion of gases in the ground
- spallation of the ground surface and two processes partially fill it back in
- fall-back of ejecta
- erosion and landslides of the crater lip and wall[31]
The relative importance of the five processes varies depending on the height above or depth below the ground surface at which the explosion occurs, and the material composing the ground.
A subsidence crater is a hole or depression left on the surface of an area which has had an underground (usually nuclear) explosion.
Subsidence craters are created as the roof of the cavity caused by the explosion collapses. This causes the surface to depress into a sink (which subsidence craters are sometimes called). It is possible for further collapse to occur from the sink into the explosion chamber. When this collapse reaches the surface, and the chamber is exposed atmospherically to the surface, it is referred to as a chimney.
When a drilling oil well encounters high-pressured gas which cannot be contained either by the weight of the drilling mud or by blow-out preventers, the resulting violent eruption can create a large crater which can swallow up a drilling rig. This phenomenon is called "cratering" in oil field slang.
An image of Tindr is shown at right. It is a pit crater.
Tindr is a crater on Jupiter's moon Callisto. It is named after one of the ancestors of Ottar in Norse mythology. This is an example of a central pit impact crater.[29]
Removal of material and rock beneath a surface may result in a collapse of material above into the cavern below.
"In accordance with its definition, a makhtesh (Hebrew for "mortar" or "crater"; plural, makhteshim) is an erosion structure incised into an anticline and having a single drainage system with one outlet."[32]
"Erosional craters (Makhtesh) were formed by truncation and erosion of several of these anticlinal crests."[33]
At lower left, the image is an oblique aerial photo of Makhtesh Hazera. The Makhtesh drainage divide is outlined by a bold black line, with both of its constituent features (the anticlinal valley and the Upper Basin) located.[33]
Bands
[edit | edit source]


In the broadest sense, the term impact crater can be applied to any depression, natural or manmade, resulting from the high velocity impact of a projectile with a larger body. In most common usage, the term is used for the approximately circular depression in the surface of a planet, moon or other solid body in the Solar System, formed by the hypervelocity impact of a smaller body with the surface. In contrast to volcanic craters, which result from explosion or internal collapse,[34] impact craters typically have raised rims and floors that are lower in elevation than the surrounding terrain.[35] Impact craters range from small, simple, bowl-shaped depressions to large, complex, multi-ringed impact basins. Meteor Crater is perhaps the best-known example of a small impact crater on the Earth.
Impact cratering involves high velocity collisions between solid objects, typically much greater than the velocity of sound in those objects. Such hyper-velocity impacts produce physical effects such as melting and vaporization that do not occur in familiar sub-sonic collisions. On Earth, ignoring the slowing effects of travel through the atmosphere, the lowest impact velocity with an object from space is equal to the gravitational escape velocity of about 11 km/s. The fastest impacts occur at more than 80 km/s in the "worst case" scenario which the asteroid hits the earth in a retrograde parabolic orbit (because kinetic energy scales as velocity squared, earth's gravity only contributes 1 km/s to this figure, not 11 km/s). The median impact velocity on Earth is about 20 to 25 km/s.
Impacts at these high speeds produce shock waves in solid materials, and both impactor and the material impacted are rapidly compressed to high density. Following initial compression, the high-density, over-compressed region rapidly depressurizes, exploding violently, to set in train the sequence of events that produces the impact crater. Impact-crater formation is therefore more closely analogous to cratering by high explosives than by mechanical displacement. Indeed, the energy density of some material involved in the formation of impact craters is many times higher than that generated by high explosives. Since craters are caused by explosions, they are nearly always circular – only very low-angle impacts cause significantly elliptical craters.[36]
The distinctive mark of an impact crater is the presence of rock that has undergone shock-metamorphic effects, such as shatter cones, melted rocks, and crystal deformations. The problem is that these materials tend to be deeply buried, at least for simple craters. They tend to be revealed in the uplifted center of a complex crater, however.
Impacts produce distinctive shock-metamorphic effects that allow impact sites to be distinctively identified. Such shock-metamorphic effects can include:
- A layer of shattered or "brecciated" rock under the floor of the crater. This layer is called a "breccia lens".
- Shatter cones, which are chevron-shaped impressions in rocks. Such cones are formed most easily in fine-grained rocks.
- High-temperature rock types, including laminated and welded blocks of sand, spherulites and tektites, or glassy spatters of molten rock. The impact origin of tektites has been questioned by some researchers; they have observed some volcanic features in tektites not found in impactites. Tektites are also drier (contain less water) than typical impactites. While rocks melted by the impact resemble volcanic rocks, they incorporate unmelted fragments of bedrock, form unusually large and unbroken fields, and have a much more mixed chemical composition than volcanic materials spewed up from within the Earth. They also may have relatively large amounts of trace elements that are associated with meteorites, such as nickel, platinum, iridium, and cobalt. Note: scientific literature has reported that some "shock" features, such as small shatter cones, which are often associated only with impact events, have been found also in terrestrial volcanic ejecta.
- Microscopic pressure deformations of minerals. These include fracture patterns in crystals of quartz and feldspar, and formation of high-pressure materials such as diamond, derived from graphite and other carbon compounds, or stishovite and coesite, varieties of shocked quartz.
- Buried craters can be identified through drill coring, aerial electromagnetic resistivity imaging, and airborne gravity gradiometry.[37]
At right is a "[r]ecent airborne geophysical surveys near Decorah, Iowa [which is] providing an unprecedented look at a 470- million-year-old meteorite crater concealed beneath bedrock and sediments."[38]
"Capturing images of an ancient meteorite impact was a huge bonus," said Dr. Paul Bedrosian, a USGS geophysicist in Denver who is leading the effort to model the recently acquired geophysical data.[38] "These findings highlight the range of applications that these geophysical methods can address."[38]
"In 2008-09, geologists from the Iowa Department of Natural Resources' (Iowa DNR) Iowa Geological and Water Survey hypothesized what has become known as the Decorah Impact Structure. The scientists examined water well drill-cuttings and recognized a unique shale unit preserved only beneath and near the city of Decorah. The extent of the shale, which was deposited after the impact by an ancient seaway, defines a "nice circular basin" of 5.5 km width, according to Robert McKay, a geologist at the Iowa Geological Survey."[38]
"Bevan French, a scientist the Smithsonian's National Museum of Natural History, subsequently identified shocked quartz - considered strong evidence of an extra-terrestrial impact - in samples of sub-shale breccia from within the crater."[38]
"The recent geophysical surveys include an airborne electromagnetic system, which is sensitive to how well rocks conduct electricity, and airborne gravity gradiometry, which measures subtle changes in rock density. The surveys both confirm the earlier work and provide a new view of the Decorah Impact Structure. Models of the electromagnetic data show a crater filled with electrically conductive shale and the underlying breccia, which is rock composed of broken fragments of rock cemented together by a fine-grained matrix."[38]
"The shale is an ideal target and provides the electrical contrast that allows us to clearly image the geometry and internal structure of the crater," Bedrosian said.[38]
The image at the right shows a crater produced by missile impact in silty sand and sandy silt photographed in an oblique view. The "[m]issile traveled along an oblique trajectory, 45.8° from the horizontal with a kinetic energy of 25.1 x 1014 ergs. The crater, about 6 metres across, and ejecta have bilateral symmetry because of the oblique trajectory. [The t]race of path of [the] missile is shown by [the] arrow. Small depressions in foreground are footprints."[39]
"Craters in natural materials at White Sands Missile Range, N. Mex., were produced by the impact of high-velocity to hypervelocity missiles traveling along oblique trajectories with kinetic energies between 2.1 and 81 x 1014 ergs. The oblique impacts produce craters 2 to 10 m across with morphologies and ejecta that are bilaterally symmetrical with respect to the plane of the missile trajectory. Rims are high and the amount of ejecta large in down-trajectory and lateral directions, whereas rims are low to nonexistent and ejecta thin to absent up-trajectory. Symmetry development and modifications of the symmetry are a function of target material, local topography, and angle of impact."[39]
Mercury
[edit | edit source]


Mercury's surface is heavily cratered and similar in appearance to Earth's Moon. For example, an unusual crater with radiating troughs has been discovered which scientists called "the spider."[40] Craters on Mercury range in diameter from small bowl-shaped cavities to multi-ringed impact basins hundreds of kilometers across. They appear in all states of degradation, from relatively fresh rayed craters to highly degraded crater remnants. Mercurian craters differ subtly from lunar craters in that the area blanketed by their ejecta is much smaller, a consequence of Mercury's stronger surface gravity.[41]
The largest known crater is Caloris Basin, with a diameter of 1,550 km.[42] The impact that created the Caloris Basin was so powerful that it caused lava eruptions and left a concentric ring over 2 km tall surrounding the impact crater. At the antipode of the Caloris Basin is a large region of unusual, hilly terrain known as the "Weird Terrain".
Overall, about 15 impact basins have been identified on the imaged part of Mercury. A notable basin is the 400 km wide, multi-ring Tolstoj Basin which has an ejecta blanket extending up to 500 km from its rim and a floor that has been filled by smooth plains materials. Beethoven Basin has a similar-sized ejecta blanket and a 625 km diameter rim.[41] Like the Moon, the surface of Mercury has likely incurred the effects of space weathering processes, including Solar wind and micrometeorite impacts.[43]
On November 29, 2012, NASA confirmed that images from the space probe Mercury Messenger had detected that craters at the north pole contained water ice.[44] Sean C. Solomon was quoted in the New York Times as estimating the volume of the ice as large enough to "encase Washington, D.C., in a frozen block two and a half miles deep."
A small crater named Hun Kal provides the reference point for measuring longitude. The center of Hun Kal is 20° west longitude.[45]
Mariner 10 provided the first close-up images of Mercury's surface, which immediately showed its heavily cratered nature
At right is an example of catena on Mercury.
Venus
[edit | edit source]
The absence of evidence of lava flow accompanying any of the visible caldera remains an enigma. The planet has few impact craters.
After the Venera missions were completed, the prime meridian was redefined to pass through the central peak in the crater Ariadne.[46][47]
Almost a thousand impact craters on Venus are evenly distributed across its surface. On Venus, about 85% of the craters are in pristine condition. Venusian craters range from 3 km to 280 km in diameter. No craters are smaller than 3 km, because of the effects of the dense atmosphere on incoming objects. Objects with less than a certain kinetic energy are slowed down so much by the atmosphere, they do not create an impact crater.[48] Incoming projectiles less than 50 meters in diameter will fragment and burn up in the atmosphere before reaching the ground.[49]
Earth
[edit | edit source]Occasionally, objects fall from the sky. When and where this occurs, depending on the energy dumped into the atmosphere and the impact on the crust of the Earth, life forms nearby hear it, feel the vibrations from it, and recoil if the intensity is too high.
Barringer Meteor Crater
[edit | edit source]


In the image at left is an aerial view of the Barringer Meteor Crater about 69 km east of Flagstaff, Arizona USA. Although similar to the aerial view of the Soudan crater, the Barringer Meteor Crater appears angular at the farthest ends rather than round.
Meteor Crater is a meteorite impact crater approximately 43 miles (69 km) east of Flagstaff, near Winslow in the northern Arizona desert of the United States. Because the US Department of the Interior Division of Names commonly recognizes names of natural features derived from the nearest post office, the feature acquired the name of "Meteor Crater" from the nearby post office named Meteor.[50] The site was formerly known as the Canyon Diablo Crater, and fragments of the meteorite are officially called the Canyon Diablo Meteorite. Scientists refer to the crater as Barringer Crater in honor of Daniel Barringer, who was first to suggest that it was produced by meteorite impact.[51]
From space the crater appears almost like a square. The image at right has a resolution of 2 meters per pixel, and illumination is from the right. Layers of exposed limestone and sandstone are visible just beneath the crater rim, as are large stone blocks excavated by the impact.
The Holsinger meteorite is the largest discovered fragment of the meteorite that created Meteor Crater and it is exhibited in the crater visitor center. The Canyon Diablo meteorite comprises many fragments of the asteroid]] that impacted at Barringer Crater (Meteor Crater), Arizona, USA. Meteorites have been found around the crater rim, and are named for nearby Canyon Diablo, which lies about three to four miles west of the crater. There are fragments in the collections of museums around the world including the Field Museum of Natural History in Chicago. The biggest fragment ever found is the Holsinger Meteorite, weighing 639 kg, now on display in the Meteor Crater Visitor Center on the rim of the crater.
Boltysh crater
[edit | edit source]


The Moderate Resolution Imaging Spectroradiometer (MODIS) on the Terra satellite captured this image of the river system on April 15, 2004. Four major rivers are shown running into the Dnieper, the large river that forms the trunk of this tree-like structure. On the far right is the Desna River, with the Seym River branching off of it. The center right branch is formed by the Sozh River and its tributaries. The center left branch is the Dnieper, and running into it from the top left is the Byarezina River. The far left branch is formed by the Prypyats River.
Complicating this tangle of flowing water, the borders of three countries snake along the rivers, sometimes following their flow, but often not. Russia is in the top right corner of the image, Ukraine forms the lower third, and Belarus is in the upper left corner. Romania, Moldova, and the Black Sea sit on the lower edge of the image.
In both images, fires are marked with red dots. The fires were likely started by farmers clearing their fields for spring planting. The false-color image, which makes the floods easier to spot, shows vegetation as green, bare ground as tan and pink, and clouds in light blue. Water is black and dark blue. In the true-color image, the landscape is still a winter brown.
The Boltysh crater or Bovtyshka crater is an impact crater in the Kirovohrad Oblast of Ukraine,[52] near the village of Bovtyshka. The crater is 24 kilometres (15 mi) in diameter and its age of 65.17 ± 0.64 million years, based on [argon-argon dating techniques, is within error of that of Chicxulub crater in Mexico and of the Cretaceous–Paleogene boundary (K–Pg boundary). The Boltysh impact likely occurred several thousand years after Chicxulub, suggesting the extinction event may have been driven by multiple meteor strikes over an extended period of time about 65 million years ago.[53] A 2021 study, however, "indicated that the strike crater did not factor into the apocalyptic die-off of the dinosaurs."[54]
Another study of ancient fern spikes suggests the Boltysh impact may have occurred several thousand years before Chicxulub.[53]
The "first direct geochronological comparison of Boltysh to the K-Pg boundary [produced a] 40Ar/39Ar age of 65.39 ± 0.14/0.16 Ma [and] shows that the impact occurred ~0.65 Ma after the mass extinction. At that time, the climate was recovering from the effects of the Chicxulub impact and Deccan trap flood volcanism."[55]
"The Boltysh impact structure, Ukraine (48°45′N; 32°10′E), is approximately 24 km in diameter with a 6-km-diameter central uplift (19). Boltysh is located in the central part of the Ukrainian Shield, where the target rocks are Precambrian granites and granitic gneisses [1550 to 2220 Ma (19)]. The structure is now buried beneath >500 m of post-impact sediments (19, 20)."[55]
Catenae
[edit | edit source]"Collisions by fragmented objects result in multiple impacts that can lead to the formation of linear crater chains, or catenae, on planetary surfaces2."[56]
Chicxulub impact crater
[edit | edit source]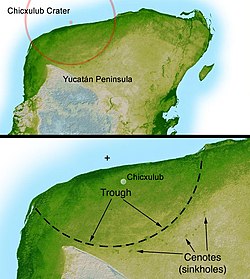
But asteroid impacts, though rare, occur once in a while, over very large areas, at Chicxulub crater. Most scientists agree that this impact is the cause of the Cretatious-Tertiary Extinction, 65 million years ago (Ma), that marked the sudden extinction of the dinosaurs and the majority of life then on Earth. This shaded relief image of Mexico's Yucatan Peninsula shows a subtle, but unmistakable, indication of the Chicxulub impact crater.
Five impact structures
[edit | edit source]"Five terrestrial impact structures have been found to possess comparable ages (214 Myr), coincident with the Norian stage of the Triassic period. These craters are Rochechouart (France), Manicouagan and Saint Martin (Canada), Obolon' (Ukraine) and Red Wing (USA). When these impact structures are plotted on a tectonic reconstruction of the North American and Eurasian plates for 214 Myr before present, the three largest structures (Rochechouart, Manicouagan and Saint Martin) are co-latitudinal at 22.8° (within 1.2°, 110 km), and span 43.5° of palaeolongitude. These structures may thus represent the remains of a crater chain at least 4,462 km long. The Obolon' and Red Wing craters, on the other hand, lie on great circles of identical declination with Rochechouart and Saint Martin, respectively. [...] the five impact structures were [likely] formed at the same time (within hours) during a multiple impact event caused by a fragmented comet or asteroid colliding with Earth."[56]
Manicouagan Impact Crater
[edit | edit source]
At right is a natural color photograph of the Aurora Borealis or northern lights and the Manicouagan Impact Crater reservoir (foreground) in Quebec, Canada. They are featured in this photograph taken by astronaut Donald R. Pettit, Expedition Six NASA ISS science officer, on board the International Space Station (ISS).
Shoemaker crater
[edit | edit source]
Shoemaker (formerly known as Teague Ring) is an impact structure, the deeply eroded remnant of a former impact crater, situated in arid central Western Australia, about 100 km (62 mi) north-northeast of Wiluna, Western Australia.[57] It is named in honour of planetary geologist Eugene Shoemaker.[58]
Tenoumer crater
[edit | edit source]
Tenoumer is considered to be an impact crater in Mauritania.[59]
The crater, located in the western Sahara Desert, is 1.9 km (1.2 mi) in diameter and its age is estimated to be 21,400 ± 9,700 years old.[60]
A volcanic origin was once theorized because of the discovery of basalt and rhyodacite outside of the crater basin,[61] but current evidence clearly indicates an impact origin.[62]
Yamal Peninsula craters
[edit | edit source]The second image down on the left shows one of the giant craters discovered on the Yamal Peninsula.
"More recently, a huge explosion was heard in June [2017] in the Yamal Peninsula. Reindeer herders camped nearby saw flames shooting up with pillars of smoke and found a large crater left in the ground. Melting permafrost was again suspected, thawing out dead vegetation and erupting in a blowout of highly flammable methane gas."[63]
"Over the past three years, 14 other giant craters have been found in the region, some of them truly massive – the first one discovered was around 50m (160ft) wide and about 70m (230ft) deep, with steep sides and debris spread all around."[63]
"The last time we saw a permafrost melting was 130,000 years ago. It's a natural phenomenon because of changes in the earth's orbit."[64]
"But what is definitely unprecedented is the rate of warming. The warming that happened 130,000 years ago happened over thousands of years … What we see happening now is warming over decades or a century."[64]
"When [permafrosts] release carbon, it will accelerate the rate of warming in the future."[64]
"All these hypotheses [on how the craters are formed], though, use the fact that temperature in the region is increasing."[65]
"There is no estimate for how much methane is released into the atmosphere because we don't know how [to measure it]."[65]
"People in permafrost regions rely on frozen ground for their infrastructure. As the ground melts, the railway collapses, the roads fall apart, the buildings sink into the ground … It's happening already."[64]
Moon
[edit | edit source]





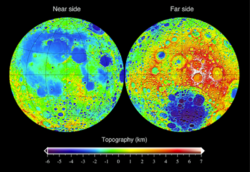




Lunar craters "are pits or depressions in the surface of the Moon, produced by great impacts of gigantic meteoroids which mostly took place billions of years ago. They range in size from huge walled plains more than a hundred miles across to microscopic pits. The smallest craters which can be glimpsed through ordinary binoculars are about twenty miles across. These craters are most common in the light-colored Lunar highlands. They are named after historical figures, mostly scientists."[66]
"During its flight, the Galileo spacecraft returned images of the Moon. The Galileo spacecraft took these images on December 7, 1992 on its way to explore the Jupiter system in 1995-97. The distinct bright ray crater at the bottom of the image is the Tycho impact basin. The dark areas are lava rock filled impact basins: Oceanus Procellarum (on the left), Mare Imbrium (center left), Mare Serenitatis and Mare Tranquillitatis (center), and Mare Crisium (near the right edge). This picture contains images through the Violet, 756 nm, 968 nm filters. The color is 'enhanced' in the sense that the CCD camera is sensitive to near infrared wavelengths of light beyond human vision."[67]
The dark irregular mare lava plains are prominent in the fully illuminated disk. A single bright star of ejecta, with rays stretching a third of the way across the disk, emblazons the lower centre: this is the crater Tycho on the Near side of the Moon. But, on the far side, the full disk is nearly featureless, a uniform grey surface with almost no dark mare. There are many bright overlapping dots of impact craters. And, an almost complete lack of dark maria.[68]
The topography of the Moon has been measured with laser altimetry [using the sodium D2 line in the yellow] and stereo image analysis.[69] The most visible topographic feature is the giant far side South Pole – Aitken basin, some 2,240 km in diameter, the largest crater on the Moon and the largest known crater in the Solar System.[70][71] At 13 km deep, its floor is the lowest elevation on the Moon.[70][72] The highest elevations are found just to its north-east, and it has been suggested that this area might have been thickened by the oblique formation impact of South Pole – Aitken.[73] Other large impact basins, such as Imbrium, Serenitatis, Crisium, Smythii, and Orientale, also possess regionally low elevations and elevated rims.[70] The lunar far side is on average about 1.9 km higher than the near side.[74]
"These images show a very young lunar crater on the side of the moon that faces away from Earth, as viewed by NASA's Moon Mineralogy Mapper [M3] on the Indian Space Research Organization's Chandrayaan-1 spacecraft. On the left is an image showing brightness at shorter infrared wavelengths. On the right, the distribution of water-rich minerals (light blue) is shown around a small crater. Both water- and hydroxyl-rich materials were found to be associated with material ejected from the crater."[75]
"Very precise microwave measurements between two spacecraft, named Ebb and Flow, were used to map gravity with high precision and high spatial resolution. The field shown [at right] resolves blocks on the surface of about 12 miles (20 kilometres) and measurements are three to five orders of magnitude improved over previous data. Red corresponds to mass excesses and blue corresponds to mass deficiencies. The map shows more small-scale detail on the far side of the moon compared to the nearside because the far side has many more small craters."[76]
"Crystallized spheres of orange glass from Shorty Crater at the Apollo 17 site are ... the characteristic ingredient of the dark mantling deposit of the Taurus-Littrow region."[77]
"A 1953 telescopic photograph of a flash on the Moon is the only unequivocal record of the rare crash of an asteroid-sized body onto the lunar surface. ... A search of images from the Clementine mission reveals an ∼1.5-km high-albedo, blue, fresh-appearing crater with an associated ejecta blanket at the location of the flash."[78]
Catena Davy is a linear string of 23 tiny craters [that] runs from the midpoint of Davy Y towards the walled basin Ptolemaeus, following a slightly curving course to the east-northeast. It is located at selenographic coordinates 11.0° S, 7.0° W, and has a diameter of 50 km. It is not radial to a suitable source crater. High resolution images have demonstrated that the craters formed at about the same time since the ejecta from each crater does not overlay neighboring craters.
Mars
[edit | edit source]


"Near the lower left corner of this view [at right] is the three-petal lander platform that NASA's Mars Exploration Rover Spirit drove off in January 2004. The lander is still bright, but with a reddish color, probably due to accumulation of Martian dust."[79]
"The High Resolution Imaging Science Experiment (HiRISE) camera on NASA's Mars Reconnaissance Orbiter recorded this view on Jan. 29, 2012, providing the first image from orbit to show Spirit's lander platform in color. The view covers an area about 2,000 feet (about 600 meters) wide, dominated by Bonneveille Crater. North is up. A bright spot on the northern edge of Bonneville Crater is a remnant of Spirit's heat shield."[79]
The shield volcano, Olympus Mons [shown in the second image at right] (Mount Olympus), at 27 km is the second highest known mountain in the Solar System.[80] It is an extinct volcano in the vast upland region Tharsis, which contains several other large volcanoes. Olympus Mons is over three times the height of Mount Everest, which in comparison stands at just over 8.8 km.[81]
Rampart craters are a specific type of Martian impact crater which are accompanied by distinctive fluidized ejecta features. A Martian Rampart crater displays an ejecta with a low ridge along its edge. Usually, rampart craters show a lobate outer margin, as if material moved along the surface, rather than flying up and down in a ballistic trajectory. The flows sometimes are diverted around small obstacles, instead of falling on them. The ejecta look as if they move as a mudflow. Some of the shapes of Rampart craters can be duplicated by shooting projectiles into mud. Although rampart craters can be found all over Mars, the smaller ones are only found in the high latitudes where ice is predicted to be close to the surface. It seems that the impact has to be powerful enough to penetrate to the level of the subsurface ice. Since ice is thought to be close to the surface in latitudes far from the equator, it does not take too strong of an impact to reach the ice level.[82] So, based on images from the Viking program in the 1970s, it is generally accepted that rampart craters are evidence of ice or liquid water beneath the surface of Mars. The impact melts or boils the water in the subsurface producing a distinctive pattern of material surrounding the crater.
Vesta
[edit | edit source]
"The [NASA's Dawn spacecraft] Framing Camera (FC) discovered enigmatic orange material on Vesta. FC images revealed diffuse orange ejecta around two impact craters, 34-km diameter Oppis, and 30-km diameter Octavia, as well as numerous sharp-edge orange units in the equatorial region."[83]
Callisto
[edit | edit source]
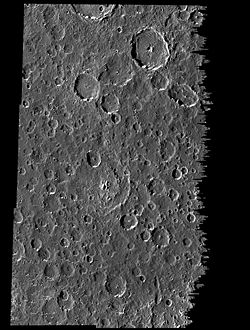

The surface of Callisto is heavily cratered and extremely old. It does not show any signatures of subsurface processes such as plate tectonics or volcanism, and is thought to have evolved predominantly under the influence of impacts.[29] Prominent surface features include multi-ring structures, variously shaped impact craters, and chains of craters (catenae) and associated scarps, ridges and deposits.[29] At a small scale, the surface is varied and consists of small, bright frost deposits at the tops of elevations, surrounded by a low-lying, smooth blanket of dark material.[84]
Many fresh impact craters like Lofn also show enrichment in carbon dioxide.[85]
The ancient surface of Callisto is one of the most heavily cratered in the Solar System.[86] In fact, the crater density is close to saturation: any new crater will tend to erase an older one. The impact craters and multi-ring structures—together with associated fractures, scarps and deposits—are the only large features to be found on the surface.[29][87]
Callisto's surface can be divided into several geologically different parts: cratered plains, light plains, bright and dark smooth plains, and various units associated with particular multi-ring structures and impact craters.[29][87] The cratered plains constitute most of the surface area and represent the ancient lithosphere, a mixture of ice and rocky material. The light plains include bright impact craters like Burr and Lofn, as well as the effaced remnants of old large craters called palimpsests, [In the case of icy satellites, palimpsests are defined as bright circular surface features, probably old impact craters; see Greeley et al. 2000.[29]] the central parts of multi-ring structures, and isolated patches in the cratered plains.[29] These light plains are thought to be icy impact deposits. The bright, smooth plains constitute a small fraction of the Callistoan surface and are found in the ridge and trough zones of the Valhalla and Asgard formations and as isolated spots in the cratered plains.
Impact crater diameters seen range from 0.1 km—a limit defined by the imaging resolution—to over 100 km, not counting the multi-ring structures.[29] Small craters, with diameters less than 5 km, have simple bowl or flat-floored shapes. Those 5–40 km across usually have a central peak. Larger impact features, with diameters in the range 25–100 km, have central pits instead of peaks, such as Tindr crater.[29] The largest craters with diameters over 60 km can have central domes, which are thought to result from central tectonic uplift after an impact;[29] examples include Doh and Hár craters. A small number of very large—more 100 km in diameter—and bright impact craters show anomalous dome geometry. These are unusually shallow and may be a transitional landform to the multi-ring structures, as with the Lofn impact feature.[29] Callistoan craters are generally shallower than those on the Moon.
The largest impact features on the Callistoan surface are multi-ring basins.[29][87] Two are enormous. Valhalla is the largest, with a bright central region 600 kilometers in diameter, and rings extending as far as 1,800 kilometers from the center (see figure).[88] The second largest is Asgard, measuring about 1,600 kilometers in diameter.[88] Multi-ring structures probably originated as a result of a post-impact concentric fracturing of the lithosphere lying on a layer of soft or liquid material, possibly an ocean.[89] The catenae—for example Gomul Catena—are long chains of impact craters lined up in straight lines across the surface. They were probably created by objects that were tidally disrupted as they passed close to Jupiter prior to the impact on Callisto, or by very oblique impacts.[29]
Europa
[edit | edit source]
The image at page top right shows the prominent crater in the lower right, Pwyll.
"This enhanced color image of the region surrounding the young impact crater Pwyll on Jupiter's moon Europa was produced by combining low resolution color data with a higher resolution mosaic of images obtained on December 19, 1996 by the Solid State Imaging (CCD) system aboard NASA's Galileo spacecraft. This region is on the trailing hemisphere of the satellite, centered at 11 degrees South and 276 degrees West, and is about 1240 kilometers across. North is toward the top of the image, and the sun illuminates the surface from the east."[90]
"The 26 kilometer diameter impact crater Pwyll, just below the center of the image, is thought to be one of the youngest features on the surface of Europa. The diameter of the central dark spot, ejecta blasted from beneath Europa's surface, is approximately 40 kilometers, and bright white rays extend for over a thousand kilometers in all directions from the impact site. These rays cross over many different terrain types, indicating that they are younger than anything they cross. Their bright white color may indicate that they are composed of fresh, fine water ice particles, as opposed to the blue and brown tints of older materials elsewhere in the image."[90]
"Also visible in this image are a number of the dark lineaments which are called "triple bands" because they have a bright central stripe surrounded by darker material. Scientists can use the order in which these bands cross each other to determine their relative ages, as they attempt to reconstruct the geologic history of Europa."[90]
Ganymede
[edit | edit source]


The image at right shows a chain of 13 craters (Enki Catena) on Ganymede measuring 161.3 km in length. The Enki craters formed across the sharp boundary between areas of bright terrain and dark terrain, delimited by a thin trough running diagonally across the center of this image. The ejecta deposit surrounding the craters appears very bright on the bright terrain. Even though all the craters formed nearly simultaneously, it is difficult to discern any ejecta deposit on the dark terrain.
Cratering is seen on both types of terrain [third image at right], but is especially extensive on the dark terrain: it appears to be saturated with impact craters and has evolved largely through impact events.[91] The brighter, grooved terrain contains many fewer impact features, which have been only of a minor importance to its tectonic evolution.[91] The density of cratering indicates an age of 4 billion years for the dark terrain, similar to the highlands of the Moon, and a somewhat younger age for the grooved terrain (but how much younger is uncertain).[92] Ganymede may have experienced a period of heavy cratering 3.5 to 4 billion years ago similar to that of the Moon.[92] If true, the vast majority of impacts happened in that epoch, while the cratering rate has been much smaller since.[93] Craters both overlay and are crosscut by the groove systems, indicating that some of the grooves are quite ancient. Relatively young craters with rays of ejecta are also visible.[93][94] Ganymedian craters are flatter than those on the Moon and Mercury. This is probably due to the relatively weak nature of Ganymede's icy crust, which can (or could) flow and thereby soften the relief. Ancient craters whose relief has disappeared leave only a "ghost" of a crater known as a palimpsest.[93]
The second image at right is an "[o]blique view of two fresh impact craters in bright grooved terrain near the north pole of Jupiter's moon, Ganymede. The craters postdate the grooved terrain since each is surrounded by swarms of smaller craters formed by material which was ejected out of the crater as it formed, and which subsequently reimpacted onto the surrounding surface. The crater to the north, Gula, which is 38 kilometers (km) in diameter, has a distinctive central peak, while the crater to the south, Achelous, (32 km in diameter) has an outer lobate ejecta deposit extending about a crater radius from the rim. Such images show the range of structural details of impact craters, and help in understanding the processes that form them."[95]
"North is to the top of the picture and the sun illuminates the surface from the right. The image, centered at 62 degrees latitude and 12 degrees longitude, covers an area approximately 142 by 132 kilometers. The resolution is 175 meters per picture element. The images were taken on April 5, 1997 at 6 hours, 33 minutes, 37 seconds Universal Time at a range of 17,531 kilometers by the Solid State Imaging (SSI) system on NASA's Galileo spacecraft."[95]
In the third image at right "[t]he ancient, dark terrain of Nicholson Regio (left) shows many large impact craters, and zones of fractures oriented generally parallel to the boundary between the dark and bright regions of Jupiter's moon Ganymede. In contrast, the bright terrain of Harpagia Sulcus (right) is less cratered and relatively smooth."[96]
"The nature of the boundary between ancient, dark terrain and younger, bright terrain, the two principal terrain types on Ganymede, was explored by NASA's Galileo spacecraft on May 20, 2000. Subtle parallel ridges and grooves show that Harpagia Sulcus's land has been smoothed out over the years by tectonic processes."[96]
"North is to the top of the picture. The Sun illuminates the surface from the left. The image, centered at ?14 degrees latitude and 319 degrees longitude, covers an area approximately 213 by 97 kilometers (132 by 60 miles.) The resolution is 121 meters (about 250 feet) per picture element. The images were taken on May 20, 2000, at a range of 11,800 kilometers (about 7,300 miles)."[96]
Io
[edit | edit source]




The dark spot just left of center [in the image at right] is the erupting volcano Prometheus. Whitish plains on either side of it are coated with volcanically emplaced sulfur dioxide frost, while yellower regions are encrusted with a higher proportion of sulfur.
Io has over 400 active volcanoes[97][98]
Several volcanoes produce plumes of sulfur and sulfur dioxide that climb as high as 500 km (300 mi) above the surface.
Seven of the nine plumes observed in March were still active in July 1979, with only the volcano Pele shutting down between flybys [of Voyager 1 then Voyager 2].[99]
Distant imaging of Io was acquired for almost every orbit during the primary mission, revealing large numbers of active volcanoes (both thermal emission from cooling magma on the surface and volcanic plumes), numerous mountains with widely varying morphologies, and several surface changes that had taken place both between the Voyager and Galileo eras and between Galileo orbits.[100] Observations during these encounters revealed the geologic processes occurring at Io's volcanoes and mountains, excluded the presence of a magnetic field, and demonstrated the extent of volcanic activity.[100] In December 2000, the Cassini spacecraft had a distant and brief encounter with the Jupiter system en route to Saturn, allowing for joint observations with Galileo. These observations revealed a new plume at Tvashtar Paterae and provided insights into Io's aurorae.[101]
New observations of Io's volcanism came from Earth-based telescopes. In particular, adaptive optics imaging from the Keck telescope in Hawaii and imaging from the Hubble telescope have allowed astronomers to monitor Io's active volcanoes.[102][103] This imaging has allowed scientists to monitor volcanic activity on Io, even without a spacecraft in the Jupiter system.
The New Horizons spacecraft, en route to Pluto and the Kuiper belt, flew by the Jupiter system and Io on February 28, 2007. During the encounter, numerous distant observations of Io were obtained. These included images of a large plume at Tvashtar, providing the first detailed observations of the largest class of Ionian volcanic plume since observations of Pele's plume in 1979.[104] New Horizons also captured images of a volcano near Girru Patera in the early stages of an eruption, and several volcanic eruptions that have occurred since Galileo.[104]
Images from the Galileo spacecraft revealed that many of Io's major lava flows, like those at Prometheus and Amirani, are produced by the build-up of small breakouts of lava flows on top of older flows.[105] Larger outbreaks of lava have also been observed on Io. For example, the leading edge of the Prometheus flow moved 75 to 95 km (47 to 59 mi) between Voyager in 1979 and the first Galileo observations in 1996. A major eruption in 1997 produced more than 3,500 km2 (1,400 sq mi) of fresh lava and flooded the floor of the adjacent Pillan Patera.[106]
The discovery of plumes at the volcanoes Pele and Loki were the first sign that Io is geologically active.[107]
The global distribution of mountains appears to be opposite that of volcanic structures; mountains dominate areas with fewer volcanoes and vice versa.[108]
Mimas
[edit | edit source]
The Herschel Crater on Saturn's moon Mimas is shown in the image at right.
Titan
[edit | edit source]
"This radar image [at right] of Titan shows a semi-circular feature that may be part of an impact crater. Very few impact craters have been seen on Titan so far, implying that the surface is young. Each new crater identified on Titan helps scientists to constrain the age of the surface."[109]
Comets
[edit | edit source]
Deep Impact is a NASA space probe launched on January 12, 2005. It was designed to study the composition of the comet interior of 9P/Tempel, by releasing an impactor into the comet. At 5:52 UTC on July 4, 2005, the impactor successfully collided with the comet's nucleus. The impact excavated debris from the interior of the nucleus, allowing photographs of the impact crater. The photographs showed the comet to be more dusty and less icy than had been expected. The impact generated a large and bright dust cloud, which unexpectedly obscured the view of the impact crater.
Hypotheses
[edit | edit source]- There is at least one key clue or ingredient that allows the differentiation of craters by their origins or causative factors.
See also
[edit | edit source]References
[edit | edit source]- ↑ 1.0 1.1 Glen A. Izett (September 26, 2000). Shocked Quartz from the USGS -- NASA Langley Core. U. S. Geological Survey. http://geology.er.usgs.gov/eespteam/crater/shockquartz.html. Retrieved 2012-10-23.
- ↑ Michael Fleischer (1962). "New mineral names" (PDF). American Mineralogist (Mineralogical Society of America) 47 (2): 172–4. http://rruff.info/uploads/AM47_805.pdf.
- ↑ R Wirth; C. Vollmer; F. Brenker; S. Matsyuk; F. Kaminsky (2007). "Inclusions of nanocrystalline hydrous aluminium silicate "Phase Egg" in superdeep diamonds from Juina (Mato Grosso State, Brazil)". Earth and Planetary Science Letters 259 (3–4): 384. doi:10.1016/j.epsl.2007.04.041.
- ↑ EP Izokh (1996). "Origin of tektites: an alternative to terrestrial impact theory". Chemie der Erde : Beitrage zur Chemischen Mineralogie, Petrographie und Geologie 56: 458-74. PMID 11541098. http://ukpmc.ac.uk/abstract/MED/11541098. Retrieved 2012-10-23.
- ↑ B. P. Glass; Jiquan Wu (May 1993). "Coesite and shocked quartz discovered in the, Australasian and North American, microtektite layers". Geology 21 (5): 435-8. doi:10.1130/0091-7613(1993)021<0435:CASQDI>2.3.CO;2. http://geology.geoscienceworld.org/content/21/5/435.short. Retrieved 2012-10-23.
- ↑ S. Marchi; W. F. Bottke; B. A. Cohen; K. Wünnemann; D. A. Kring; H. Y. McSween; M. C. De Sanctis; D. P. O’Brien et al. (March 2013). "High-velocity collisions from the lunar cataclysm recorded in asteroidal meteorites". Nature Geoscience 6 (4): 303-7. doi:10.1038/ngeo1769. http://www.nature.com/ngeo/journal/v6/n4/full/ngeo1769.html. Retrieved 2013-03-29.
- ↑ Apollo over the Moon - Chapter 5: Craters. http://www.hq.nasa.gov/office/pao/History/SP-362/ch5.3.htm. Retrieved 2008-02-03.
- ↑ J.L. Jordan; J.R. Walton; D. Heymann; S. Lakatos (March 1974). "The Rim of North Ray Crater: A Relatively Young Regolith". Abstracts of the Lunar and Planetary Science Conference 5 (03): 388. http://adsabs.harvard.edu/abs/1974LPI.....5..388J. Retrieved 2013-10-18.
- ↑ 9.0 9.1 Warren Wiscombe (July 19, 2009). Mount Tambora Volcano, Sumbawa Island, Indonesia. NASA Earth Observatory. http://earthobservatory.nasa.gov/IOTD/view.php?id=39412. Retrieved 2013-03-30.
- ↑ Glossary of Terms: C. physicalgeography.net. http://www.physicalgeography.net/physgeoglos/c.html. Retrieved 2008-04-12.
- ↑ Susan S. Priest; Wendell A. Duffield; Karen Malis-Clark; James W. Hendley II; Peter H. Stauffer (2001-12-21). The San Francisco Volcanic Field, Arizona: USGS Fact Sheet 017-01. United States Geological Survey. http://geopubs.wr.usgs.gov/fact-sheet/fs017-01/. Retrieved 2008-09-02.
- ↑ Rosaly Lopes (2005-02-07). The Volcano Adventure Guide. Cambridge University Press. pp. 153. ISBN 978-0-521-55453-4. http://books.google.com/?id=eRqrEwvIvKoC&pg=PA153&lpg=PA153&dq=SP+Crater.
- ↑ 13.0 13.1 Ulrich, G E (1987). SP Mountain cinder cone and lava flow, northern Arizona. Geological Society of American Centennial Field Guide – Rocky Mountain Section. pp. 385–8.
- ↑ Basksi A, K (1974). "K-Ar study of the S.P. flow". Canadian Journal of Earth Sciences 11: 1350–1356.
- ↑ Duffield, Wendell A; Riggs, Nancy; Kaufman, Darrell; Champion, Duane; Fenton, Cassandra; Forman, Steven; McIntosh, William; Hereford, Richard et al. (2006). "Multiple constraints on the age of a Pleistocene lava dam across the Little Colorado River at Grand Falls, Arizona". Geological Society of America Bulletin 118 (3-4): 421-9.
- ↑ A.R. Hildebrand; J.A. Stansberry (March 1992). "K/T boundary ejecta distribution predicts size and location of Chicxulub crater". Abstracts of the Lunar and Planetary Science Conference 23 (03): 537. http://adsabs.harvard.edu/full/1992LPI....23..537H. Retrieved 2013-10-18.
- ↑ P. Ma; K. Aggrey; C. Tonzola; C. Schnabel; P. de Nicola; G.F. Herzog; J.T. Wasson; B.P. Glass et al. (October 2004). "Beryllium-10 in Australasian tektites: constraints on the location of the source crater". Geochimica et Cosmochimica Acta 68 (19): 3883-96. http://www.sciencedirect.com/science/article/pii/S0016703704002741. Retrieved 2013-10-18.
- ↑ E. M. Shoemaker (1977). Astronomically observable crater-forming projectiles, In: Impact and Explosion Cratering: Planetary and terrestrial implications. New York: Pergamon Press, Inc.. pp. 617-28. http://adsabs.harvard.edu/abs/1977iecp.symp..617S. Retrieved 2013-10-18.
- ↑ C. C. Porco1; E. Baker; J. Barbara; K. Beurle; A. Brahic; J. A. Burns; S. Charnoz; N. Cooper et al. (February 2005). "Cassini imaging science: Initial results on Phoebe and Iapetus". Science 307 (5713): 1237-42. http://www.sciencemag.org/content/307/5713/1237.short. Retrieved 2013-10-18.
- ↑ Stanley Ambrose page at University of Illinois at Urbana-Champaign
- ↑ Supervolcanoes, BBC2, 3 February 2000
- ↑ Gazetteer of Planetary Nomenclature - Descriptor Terms (Feature Types). http://planetarynames.wr.usgs.gov/jsp/append5.jsp. Retrieved 2008-02-03.
- ↑ Harold Masursky; G. W. Colton; Farouk El-Baz (December 1978). APOLLO OVER THE MOON: A VIEW FROM ORBIT (NASA SP-362), Cahpter 5: Craters (3/6). Washington, DC USA: NASA Headquarters. http://www.hq.nasa.gov/office/pao/History/SP-362/ch5.3.htm. Retrieved 2013-03-31.
- ↑ 24.00 24.01 24.02 24.03 24.04 24.05 24.06 24.07 24.08 24.09 24.10 Mel Acheson; Amy Acheson (2005). J. Douglas Kenyon. ed. Thunderbolts of the Gods: Does Growing Evidence of an Electric Universe Reveal Previously Hidden Meaning in ancient Mythology?, In: Forbidden History Prehistoric Technologies, Extraterrestrial Intervention, and the Suppressed Origins of Civilization. Rochester, Vermont: Bear & Company. pp. 69-77. http://books.google.com/books?hl=en&lr=&id=DBDe-vhGTR0C&oi=fnd&pg=PP1&ots=CIEnZrYfQI&sig=4waK0rG-TNNQwTqCAT9FpPp3WLI#v=onepage&f=false. Retrieved 2012-06-12.
- ↑ C. J. Ransom (2011). "Laboratory Modeling of Meteorite Impact Craters by Z-pinch Plasma". The Open Astronomy Journal 4 (Suppl 2-M4): 185-90. http://www.benthamscience.com/open/toaaj/articles/V004/SI0162TOAAJ/185TOAAJ.pdf. Retrieved 2013-10-18.
- ↑ Christopher C. Burt, Extreme Weather: A Guide & Record Book (W. W. Norton & Company, 2007), p149
- ↑ B.K. Lucchitta; H.M. Ferguson (March 1988). "Ganymede: "Moat" Craters Compared with Palimpsests and Basins". Abstracts of the Lunar and Planetary Science Conference 19 (03): 701. http://adsabs.harvard.edu/full/1988LPI....19..701L. Retrieved 2013-10-18.
- ↑ Sublette, Carey. The Effects of Underground Explosions. http://nuclearweaponarchive.org/Library/Effects/UndergroundEffects.html. Retrieved 21 June 2011.
- ↑ 29.00 29.01 29.02 29.03 29.04 29.05 29.06 29.07 29.08 29.09 29.10 29.11 29.12 29.13 Greeley, R.; Klemaszewski, J.E.;Wagner L. (2000). "Galileo views of the geology of Callisto". Planetary and Space Science 48 (9): 829–853. doi:10.1016/S0032-0633(00)00050-7.
- ↑ 30.0 30.1 30.2 30.3 30.4 Kerry Sieh (January 31, 2012). Long Valley Caldera Field Guide - Panum Crater. USGS. http://volcanoes.usgs.gov/volcanoes/long_valley/long_valley_sub_page_17.html. Retrieved 2013-03-30.
- ↑ P. W. Cooper Explosives Engineering Wiley-VCH ISBN 0-471-18636-8
- ↑ Gregory Insarov; Irina Insarova (1995). "The lichens of calcareous rocks in the Central Negev, Israel". Israel Journal of Plant Sciences 43 (1): 53-62. doi:10.1080/07929978.1995.10676590. http://www.tandfonline.com/doi/abs/10.1080/07929978.1995.10676590. Retrieved 2013-10-16.
- ↑ 33.0 33.1 N. Fruchter; A. Matmon; Y. Avni; D. Fink (November 15, 2011). "Revealing sediment sources, mixing, and transport during erosional crater evolution in the hyperarid Negev Desert, Israel". Geomorphology 134 (3-4): 363-77. http://www.sciencedirect.com/science/article/pii/S0169555X11003564. Retrieved 2013-10-16.
- ↑ Basaltic Volcanism Study Project. (1981). Basaltic Volcanism on the Terrestrial Planets; Pergamon Press, Inc: New York, p. 746. http://articles.adsabs.harvard.edu//full/book/bvtp./1981//0000746.000.html.
- ↑ Consolmagno, G.J.; Schaefer, M.W. (1994). Worlds Apart: A Textbook in Planetary Sciences; Prentice Hall: Englewood Cliffs, NJ, p.56.
- ↑ Melosh, H.J., 1989, Impact cratering: A geologic process: New York, Oxford University Press, 245 p.
- ↑ US Geological Survey. Iowa Meteorite Crater Confirmed. http://www.usgs.gov/newsroom/article.asp?ID=3521. Retrieved 7 March 2013.
- ↑ 38.0 38.1 38.2 38.3 38.4 38.5 38.6 Heidi Koontz; Robert McKay (March 5, 2013). Iowa Meteorite Crater Confirmed. Reston, Virginia, USA: U.S. Geological Survey. http://www.usgs.gov/newsroom/article.asp?ID=3521#.UVfS467Qorc. Retrieved 2013-03-30.
- ↑ 39.0 39.1 Henry J. Moore (1976). Missile impact craters (White Sands Missile Range, New Mexico) and applications to lunar research: Contributions to astrogeology. Washington, DC USA: USGS. http://pubs.usgs.gov/pp/0812b/report.pdf. Retrieved 2014-06-13.
- ↑ Staff (February 28, 2008). Scientists see Mercury in a new light. Science Daily. http://www.sciencedaily.com/releases/2008/02/080201093149.htm. Retrieved 2008-04-07.
- ↑ 41.0 41.1 Spudis, P. D. (2001). "The Geological History of Mercury". Workshop on Mercury: Space Environment, Surface, and Interior, Chicago: 100.
- ↑ Shiga, David (January 30, 2008). Bizarre spider scar found on Mercury's surface. NewScientist.com news service. http://space.newscientist.com/article/dn13257-bizarre-spider-scar-found-on-mercurys-surface.html.
- ↑ Denevi, B. W.; Robinson; Robinson, M. S. (2008). "Albedo of Immature Mercurian Crustal Materials: Evidence for the Presence of Ferrous Iron". Lunar and Planetary Science 39: 1750.
- ↑ Kenneth Chang (2012-11-29). On Closest Planet to the Sun, NASA Finds Lots of Ice. New York Times. p. A3. https://www.nytimes.com/2012/11/30/science/space/mercury-home-to-ice-messenger-spacecraft-findings-suggest.html. Retrieved 2012-11-29.
- ↑ USGS Astrogeology: Rotation and pole position for the Sun and planets (IAU WGCCRE). http://astrogeology.usgs.gov/Projects/WGCCRE/constants/iau2000_table1.html. Retrieved 22 October 2009.
- ↑ USGS Astrogeology: Rotation and pole position for the Sun and planets (IAU WGCCRE). http://astrogeology.usgs.gov/Projects/WGCCRE/constants/iau2000_table1.html. Retrieved 22 October 2009.
- ↑ The Magellan Venus Explorer's Guide. http://www2.jpl.nasa.gov/magellan/guide8.html. Retrieved 22 October 2009.
- ↑ R. R. Herrick; R. J. Phillips (1993). "Effects of the Venusian atmosphere on incoming meteoroids and the impact crater population". Icarus 112 (1): 253–281. doi:10.1006/icar.1994.1180.
- ↑ David Morrison (2003). The Planetary System. Benjamin Cummings. ISBN 0-8053-8734-X.
- ↑ J. P. Barringer's acceptance speech. Meteoritics, volume 28, page 9 (1993). Retrieved on the SAO/NASA Astrophysics Data System
- ↑ Grieve, R.A.F. (1990) Impact Cratering on the Earth, Scientific American 262(4), 66–73.
- ↑ Boltysh at EID
- ↑ 53.0 53.1 "Double meteorite strike 'caused dinosaur extinction". BBC News. 2010-08-27. Retrieved 2010-08-27.
- ↑ "A Mysterious Craters Age May Add Clues to the Dinosaur Extinction".
- ↑ 55.0 55.1 Annemarie E. Pickersgill, Darren F. Mark, Martin R. Lee, Simon P. Kelley and David W. Jolley (18 June 2021). "The Boltysh impact structure: An early Danian impact event during recovery from the K-Pg mass extinction". Sciences Advances 7 (25): eabe6530. doi:10.1126/sciadv.abe6530. https://advances.sciencemag.org/content/7/25/eabe6530. Retrieved 18 June 2021.
- ↑ 56.0 56.1 John G. Spray; Simon P. Kelley; David B. Rowley (12 March 1998). "Evidence for a late Triassic multiple impact event on Earth". Nature 392 (6672): 171-3. doi:10.1038/32397. http://www.nature.com/nature/journal/v392/n6672/abs/392171a0.html. Retrieved 2014-08-06.
- ↑ "Shoemaker". Earth Impact Database. Planetary and Space Science Centre University of New Brunswick Fredericton. Retrieved 2017-10-09.
- ↑ Pirajno F. & Glikson A.Y. 1998. Shoemaker impact structure Western Australia (formerly Teague ring structure). Celestial Mechanics and Dynamical Astronomy 69, 25–30.
- ↑ "Tenoumer". Earth Impact Database. Planetary and Space Science Centre University of New Brunswick Fredericton. Retrieved 2009-08-16.
- ↑ Schultze, Dina Simona; Jourdan, Fred; Hecht, Lutz; Reimold, Wolf Uwe; Ralf-Thomas, Schmitt (2016). "Tenoumer impact crater, Mauritania: Impact melt genesis from a lithologically diverse target". Meteoritics and Planetary Science (The Meteoritical Society) 51 (2): 323–350. doi:10.1111/maps.12593. http://www.lpi.usra.edu/meetings/lpsc2003/pdf/1183.pdf.
- ↑ METEORITE OR VOLCANO?
- ↑ Brügge, Norbert. "The "impact" craters of Mauritania: Aouelloul, Tenoumer, Temimichat and El Mrayer". Archived from the original on 2010-02-23.
- ↑ 63.0 63.1 Jeremy Plester (20 July 2017). All hell breaks loose as the tundra thaws. The Guardian. https://www.theguardian.com/environment/2017/jul/20/hell-breaks-loose-tundra-thaws-weatherwatch#img-1. Retrieved 2017-07-31.
- ↑ 64.0 64.1 64.2 64.3 Gideon Henderson (30 July 2017). Mysterious craters blowing out of Russia could mean trouble for the whole planet. Yahoo News. https://www.yahoo.com/news/m/da88ff71-92f7-3f97-a8c7-705d13f80680/mysterious-craters-blowing.html?.tsrc=daily_mail&uh_test=2_04. Retrieved 2017-07-31.
- ↑ 65.0 65.1 Vladimir Romanovsky (30 July 2017). Mysterious craters blowing out of Russia could mean trouble for the whole planet. Yahoo News. https://www.yahoo.com/news/m/da88ff71-92f7-3f97-a8c7-705d13f80680/mysterious-craters-blowing.html?.tsrc=daily_mail&uh_test=2_04. Retrieved 2017-07-31.
- ↑ Fountains of Bryn Mawr (October 30, 2008). Skygazing. http://en.wikiversity.org/w/index.php?title=Skygazing&oldid=367815. Retrieved 2013-03-30.
- ↑ Jon Nelson (June 8, 1998). Earth's Moon. NASA/JPL/USGS. http://www.jpl.nasa.gov/spaceimages/details.php?id=PIA00405. Retrieved 2012-09-26.
- ↑ Landscapes from the ancient and eroded lunar far side. esa. http://www.esa.int/esaSC/SEMDWNWALPE_index_0.html. Retrieved 15 February 2010.
- ↑ Spudis, Paul D.; Cook; Robinson; Bussey; Fessler (01/1998). "Topography of the South Polar Region from Clementine Stereo Imaging". Workshop on New Views of the Moon: Integrated Remotely Sensed, Geophysical, and Sample Datasets: 69.
- ↑ 70.0 70.1 70.2 Spudis, Paul D.; Reisse, Robert A.; Gillis, Jeffrey J. (1994). "Ancient Multiring Basins on the Moon Revealed by Clementine Laser Altimetry". Science 266 (5192): 1848–1851. doi:10.1126/science.266.5192.1848. PMID 17737079.
- ↑ Pieters, C.M.; Tompkins, S.; Head, J.W.; Hess, P.C. (1997). "Mineralogy of the Mafic Anomaly in the South Pole‐Aitken Basin: Implications for excavation of the lunar mantle". Geophysical Research Letters 24 (15): 1903–6. doi:10.1029/97GL01718. http://www.agu.org/pubs/crossref/1997/97GL01718.shtml.
- ↑ Taylor, G.J. (17 July 1998). The Biggest Hole in the Solar System. Planetary Science Research Discoveries, Hawai'i Institute of Geophysics and Planetology. http://www.psrd.hawaii.edu/July98/spa.html. Retrieved 12 April 2007.
- ↑ Schultz, P. H. (March 1997). "Forming the south-pole Aitken basin – The extreme games". Conference Paper, 28th Annual Lunar and Planetary Science Conference 28: 1259.
- ↑ Wieczorek, M. et al. (2006). "The constitution and structure of the lunar interior". Reviews in Mineralogy and Geochemistry 60 (1): 221–364. doi:10.2138/rmg.2006.60.3.
- ↑ Jim Wilson (September 24, 2009). Water Around a Fresh Crater. NASA. http://www.nasa.gov/topics/moonmars/features/clark3.html. Retrieved 2012-09-26.
- ↑ Tony Greicius (December 6, 2012). GRAIL's Gravity Map of the Moon. NASA/JPL-Caltech/MIT/GSFC. http://www.nasa.gov/mission_pages/grail/multimedia/zuber4.html. Retrieved 2012-12-15.
- ↑ John B. Adams; Carle Pieters; Thomas B. McCord (March 18-22 1974). Orange glass: Evidence for regional deposits of pyroclastic origin on the moon, In: Proceedings of the Fifth Lunar Science Conference. 1. New York: Pergamon Press, Inc.. pp. 171-86. http://adsabs.harvard.edu//abs/1974LPSC....5..171A. Retrieved 2012-11-02.
- ↑ Bonnie J Buratti; Lane L Johnson (January 2003). "Identification of the lunar flash of 1953 with a fresh crater on the moon’s surface". Icarus 161 (1): 192-7. doi:10.1016/S0019-1035(02)00027-1. http://www.sciencedirect.com/science/article/pii/S0019103502000271. Retrieved 2012-11-27.
- ↑ 79.0 79.1 HiRise (February 8, 2012). PIA15038: Spirit Lander and Bonneville Crater in Color. Pasadena, California USA: NASA/JPL. http://photojournal.jpl.nasa.gov/catalog/PIA15038. Retrieved 2013-03-31.
- ↑ Craig Glenday (2009). Guinness World Records. Random House, Inc.. p. 12. ISBN 0-553-59256-4.
- ↑ Junyong Chen (2006). "Progress in technology for the 2005 height determination of Qomolangma Feng (Mt. Everest)". Science in China Series D: Earth Sciences 49 (5): 531–8. doi:10.1007/s11430-006-0531-1.
- ↑ Hugh H. Kieffer (1992). Mars. University of Arizona Press. ISBN 978-0-8165-1257-7. http://books.google.com/books?id=NoDvAAAAMAAJ. Retrieved 7 March 2011.
- ↑ L Le Corre; V Reddy; KJ Becker (October 2012). "Nature of Orange Ejecta Around Oppia and Octavia Craters on Vesta from Dawn Framing Camera". American Astronomical Society, DPS meeting (44).
- ↑ Moore, Jeffrey M., Chapman, Clark R.; Bierhaus, Edward B. (2004). Bagenal, F.. ed. Callisto, In: Jupiter: The planet, Satellites and Magnetosphere. Cambridge University Press. http://lasp.colorado.edu/~espoclass/homework/5830_2008_homework/Ch17.pdf.
- ↑ Hibbitts, C.A., McCord, T. B.; Hansen, G.B. (1998). Distributions of CO2 and SO2 on the Surface of Callisto. Lunar and Planetary Science XXXI. p. 1908. http://www.lpi.usra.edu/meetings/lpsc2000/pdf/1908.pdf.
- ↑ Zahnle, K.; Dones, L. (1998). "Cratering Rates on the Galilean Satellites". Icarus 136 (2): 202–222. doi:10.1006/icar.1998.6015. PMID 11878353. http://lasp.colorado.edu/icymoons/europaclass/Zahnle_etal_1998.pdf.
- ↑ 87.0 87.1 87.2 Bender, K. C.; Rice, J. W.; Wilhelms, D. E.; Greeley, R. (1997). Geological map of Callisto. U.S. Geological Survey. http://astrogeology.usgs.gov/Projects/PlanetaryMapping/DIGGEOL/galsats/callisto/jcglobal.htm.
- ↑ 88.0 88.1 Controlled Photomosaic Map of Callisto JC 15M CMN (2002 ed.). U.S. Geological Survey. http://geopubs.wr.usgs.gov/i-map/i2770/.
- ↑ Klemaszewski, J.A., Greeley, R. (2001). Geological Evidence for an Ocean on Callisto. Lunar and Planetary Science XXXI. p. 1818. http://www.lpi.usra.edu/meetings/lpsc2001/pdf/1818.pdf.
- ↑ 90.0 90.1 90.2 Sue Lavoie (March 6, 1998). PIA01211: Pwyll Crater on Europa. Washington, DC USA: NASA's Office of Space Science. http://photojournal.jpl.nasa.gov/catalog/PIA01211. Retrieved 2013-06-24.
- ↑ 91.0 91.1 Adam P. Showman; Renu Malhotra (1999). "The Galilean Satellites". Science 286 (5437): 77–84. doi:10.1126/science.286.5437.77. PMID 10506564. http://www.lpl.arizona.edu/~showman/publications/showman-malhotra-1999.pdf.
- ↑ 92.0 92.1 K. Zahnle; L. Dones (1998). "Cratering Rates on the Galilean Satellites". Icarus 136 (2): 202–22. doi:10.1006/icar.1998.6015. PMID 11878353. http://lasp.colorado.edu/icymoons/europaclass/Zahnle_etal_1998.pdf.
- ↑ 93.0 93.1 93.2 Ganymede. nineplanets.org. October 31, 1997. http://www.nineplanets.org/ganymede.html. Retrieved 2008-02-27.
- ↑ Ganymede. Lunar and Planetary Institute. 1997. http://www.lpi.usra.edu/resources/outerp/gany.html.
- ↑ 95.0 95.1 Sue Lavoie (July 15, 1998). PIA01609: Fresh Impact Craters on Ganymede. Washington, DC USA: NASA's Office of Space Science. http://photojournal.jpl.nasa.gov/catalog/PIA01609. Retrieved 2013-06-22.
- ↑ 96.0 96.1 96.2 Sue Lavoie (December 16, 2000). PIA02577: Bright-Dark terrain boundary, Ganymede. Washington, DC USA: NASA's Office of Space Science. http://photojournal.jpl.nasa.gov/catalog/PIA02577. Retrieved 2013-06-22.
- ↑ Rosaly MC Lopes (2006). "Io: The Volcanic Moon". In Lucy-Ann McFadden. Encyclopedia of the Solar System. Academic Press. pp. 419–431. ISBN 978-0-12-088589-3.
- ↑ R. M. C. Lopes (2004). "Lava lakes on Io: Observations of Io’s volcanic activity from Galileo NIMS during the 2001 fly-bys". Icarus 169 (1): 140–74. doi:10.1016/j.icarus.2003.11.013.
- ↑ R. G. Strom; Schneider N. M. (1982). Morrison, D.. ed. Volcanic eruptions on Io, In: Satellites of Jupiter. University of Arizona Press. pp. 598–633. ISBN 0-8165-0762-7.
- ↑ 100.0 100.1 Perry, J. (2007). Lopes, R. M. C.. ed. A Summary of the Galileo mission and its observations of Io, In: Io after Galileo. Springer-Praxis. pp. 35–59. ISBN 3-540-34681-3.
- ↑ C. C. Porco (2003). "Cassini imaging of Jupiter's atmosphere, satellites, and rings". Science 299 (5612): 1541–1547. doi:10.1126/science.1079462. PMID 12624258.
- ↑ Marchis, F. (2005). "Keck AO survey of Io global volcanic activity between 2 and 5 μm". Icarus 176 (1): 96–122. doi:10.1016/j.icarus.2004.12.014.
- ↑ Spencer, John (2007-02-23). Here We Go!. http://planetary.org/blog/article/00000874/. Retrieved 2007-06-03.
- ↑ 104.0 104.1 Spencer, J. R. (2007). "Io Volcanism Seen by New Horizons: A Major Eruption of the Tvashtar Volcano". Science 318 (5848): 240–243. doi:10.1126/science.1147621. PMID 17932290.
- ↑ Keszthelyi, L. (2001). "Imaging of volcanic activity on Jupiter's moon Io by Galileo during the Galileo Europa Mission and the Galileo Millennium Mission". J. Geophys. Res. 106 (E12): 33025–33052. doi:10.1029/2000JE001383.
- ↑ McEwen, A. S. (1998). "High-temperature silicate volcanism on Jupiter's moon Io". Science 281 (5373): 87–90. doi:10.1126/science.281.5373.87. PMID 9651251.
- ↑ Morabito, L. A. (1979). "Discovery of currently active extraterrestrial volcanism". Science 204 (4396): 972. doi:10.1126/science.204.4396.972. PMID 17800432.
- ↑ McKinnon, W. B. (2001). "Chaos on Io: A model for formation of mountain blocks by crustal heating, melting, and tilting". Geology 29 (2): 103–106. doi:10.1130/0091-7613(2001)029<0103:COIAMF>2.0.CO;2.
- ↑ Sue Lavoie (February 26, 2007). PIA09175: A New Crater on Titan?. Pasadena, California USA: NASA/JPL. http://photojournal.jpl.nasa.gov/catalog/PIA09175. Retrieved 2013-06-13.
External links
[edit | edit source]- International Astronomical Union
- NASA/IPAC Extragalactic Database - NED
- NASA's National Space Science Data Center
- Office of Scientific & Technical Information
- The SAO/NASA Astrophysics Data System
- SDSS Quick Look tool: SkyServer
- SIMBAD Astronomical Database
- SIMBAD Web interface, Harvard alternate
- Spacecraft Query at NASA
- Universal coordinate converter
Learn more about Craters |